This chapter should be cited as follows:
Bardají A, Mayor A, et al, Glob. libr. women's med.,
ISSN: 1756-2228; DOI 10.3843/GLOWM.419503
The Continuous Textbook of Women’s Medicine Series – Obstetrics Module
Volume 17
Maternal immunization
Volume Editors:
Professor Asma Khalil, The Royal College of Obstetricians and Gynaecologists, London, UK; Fetal Medicine Unit, Department of Obstetrics and Gynaecology, St George’s University Hospitals NHS Foundation Trust, London, UK
Professor Flor M Munoz, Baylor College of Medicine, TX, USA
Professor Ajoke Sobanjo-ter Meulen, University of Washington, Seattle, WA, USA
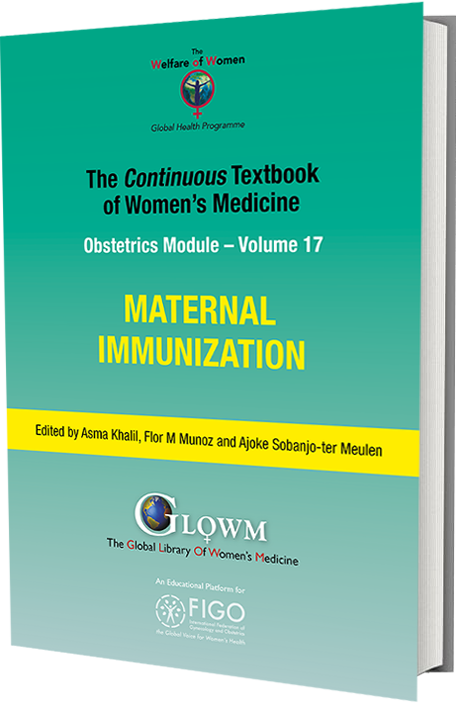
Chapter
Vaccines to Prevent Malaria in Pregnancy
First published: May 2023
Study Assessment Option
By completing 4 multiple-choice questions (randomly selected) after studying this chapter readers can qualify for Continuing Professional Development awards from FIGO plus a Study Completion Certificate from GLOWM
See end of chapter for details
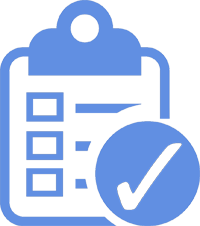
INTRODUCTION
Malaria in pregnancy: a maternal and infant health problem
An estimated 125 million pregnancies occur every year in countries with ongoing malaria transmission.1 Pregnant women are more susceptible to the effects of malaria infection. Both pregnancy-specific immunological responses and malaria-specific interactions, such as sequestration of parasites in the placenta, might contribute to this susceptibility.2,3 The latter results in an augmented risk of morbidity in the mother, mainly anemia, and low birth weight and prematurity in the infant; it is also associated with increased maternal and neonatal and infant mortality through both direct and indirect mechanisms.4,5,6 Owing to these harmful effects, malaria in pregnancy is a significant driver of maternal and neonatal health in endemic areas.
Important progress has been made in recent years, especially in sub-Saharan Africa, with the introduction of strategies to prevent malaria in pregnancy consisting primarily of administration of intermittent preventive treatment during pregnancy with an antimalarial drug and the use of long-lasting insecticide-treated nets.7 However, malaria continues to exact a huge toll on pregnant women and their newborns,8 as the effectiveness of control strategies is hampered by limitations in their implementation, with coverage still far below the target of universal access. This situation has driven the need to explore other preventive strategies that could overcome these problems, such as vaccines to prevent malaria in pregnancy. These can potentially be an important tool that, in combination with the current tools, would maximize protection and thereby control the deleterious effects of malaria in pregnancy on the health of mothers and children.
EPIDEMIOLOGY AND BURDEN OF MALARIA IN PREGNANCY
“So long as woman has walked the earth, malaria may have stalked her”.9 Malaria is the most important parasitic infection of humans and a scourge for millennia, but the burden of malaria infection in pregnancy and the detrimental effects on the health of mothers and their infants were not described in detail until early in the 20th century.10,11
Globally, in 2020 there were an estimated 241 million malaria cases in 85 malaria endemic countries and 627,000 malaria deaths.8 Of the five Plasmodium species that cause human malaria (Plasmodium falciparum, vivax, ovale, malariae, and knowlesi), P. falciparum is responsible for the most deaths and morbidity,8 and its greatest burden concentrates in areas of stable transmission in sub-Saharan Africa.
In 2020, in 33 moderate to high transmission countries in the World Health Organization (WHO) Africa region, there were an estimated 33.8 million pregnancies, of which 11.6 million (34%) were exposed to malaria infection during pregnancy.8 P. falciparum is uniquely capable to adhere and sequester in the placenta, a feature that is considered to be central to most of the manifestations of P. falciparum malaria in pregnancy.12 Pregnant women are more susceptible to the effects of malaria infection caused by both P. falciparum and P. vivax, which might be explained by pregnancy-specific immunological responses and parasite-specific effects including placental sequestration (especially in the case of P. falciparum).2,3 Malaria susceptibility in pregnancy is modulated by maternal age, parity, gestational age, the level of malaria-specific acquired immunity and HIV infection. In high-transmission areas, malaria risk is highest in the second and third trimesters of pregnancy,4,13 and the risk does not immediately return to pre-pregnancy levels after delivery.5 Malaria in pregnancy is more common in younger compared to older pregnant women, in women who are pregnant for the first time or second time (primigravidae or secundigravidae) compared with subsequent pregnancies (where adverse effects are ameliorated by antibodies that block the adhesion of P. falciparum parasitized red blood cells to chondroitin sulphate A in the placenta),14 and in women with HIV infection compared with HIV-uninfected women.4,15 Similar to the non-pregnant population, malaria risk in pregnancy is affected by location (increased risk in rural compared to urban/peri-urban areas), season, and use of malaria preventive strategies.5
Malaria and HIV continue to be major global problems with devastating consequences on the health of mothers and their babies.8,16 A considerable proportion of African pregnant women are HIV-infected, with over 12 million HIV-infected women of reproductive age exposed to malaria and more than one million pregnancies per year co-infected with malaria and HIV in this region.16,17 HIV-infected pregnant women are at an increased risk of malaria infection, including placental malaria, higher parasitemia, and severe anemia, which translates into a higher risk of maternal mortality, prematurity, and low birth weight (LBW).18 HIV infection alters the partial parity-associated antimalarial immunity thought to protect multigravidae women from adverse malaria-related pregnancy outcomes; leading to increased risk of malaria in HIV-infected multigravidae.18
Pregnant women also have increased susceptibility to P. vivax malaria. Most pregnancies at risk of vivax malaria occur in low malaria transmission areas in the Asia-Pacific region, followed by the American region. The prevalence of P. vivax infection in pregnant women was 0.4% by microscopy and 7% by polymerase chain reaction (PCR) technique in a large multicountry study of the burden and impact of P. vivax in pregnancy.19 Rosetting of infected red cells with uninfected red cells is a frequent cytoadhesive phenotype in P. vivax infections, though other adhesive features are not prominent in this species.20
CONSEQUENCES ON MATERNAL AND INFANT HEALTH
Effect on maternal health
In areas of high and stable transmission, pregnant women have a higher risk of malaria infection and greater parasitemia density compared to non-pregnant women.4 Though many malaria infections in these areas are asymptomatic due to pre-existing malaria-specific acquired immunity, the increased parasitemic burden is associated with higher risk of clinical malaria in pregnancy.4,13 Anemia is the most common outcome associated to P. falciparum malaria infection, and a risk factor for maternal death and poor infant outcomes. In sub-Saharan Africa, it has been estimated that P. falciparum malaria in pregnancy is the primary cause of up to 10,000 maternal anemia-related deaths annually.21
Maternal mortality is a deplorable problem in sub-Saharan Africa. While obstetric complications are considered the most common causes of maternal death, infectious etiologies have been increasingly recognized as an important factor in maternal mortality. P. falciparum malaria has been estimated to account for 10–20% of maternal mortality in malaria endemic countries in hospital studies,22 which was confirmed by a full-autopsy study of maternal mortality showing that malaria directly contributed to 10% of maternal deaths.23
In areas of low and unstable malaria transmission, where P. vivax is the predominant species, women have little acquired immunity and are therefore more likely to be symptomatic and to suffer severe disease with high case fatality rates.4 Studies investigating the effects of P. vivax infection in pregnancy on the health of mothers in these areas showed that it is associated with increased risk of maternal anemia and clinical malaria.5,19,24,25
Effect on fetal and infant health
In high-transmission endemic areas, malaria in pregnancy increases the risk of fetal loss (miscarriage and stillbirth),26 preterm delivery (PTD), neonatal mortality and LBW.27 Fetal growth restriction (FGR) is associated to placental parasitization and an underlying cause of most adverse effects of malaria in pregnancy.12 The main effect of malaria in pregnancy that impacts the health of the baby is LBW, due to PTD and/or FGR. In 2020, in 33 endemic countries in WHO African region, malaria infection during pregnancy resulted in an estimated 819,000 children with LBW, with 54.1% of these children from the subregion of West Africa.8 Also, LBW is associated with a significant increase in neonatal and infant mortality, with 5–8% of all infant deaths attributable to LBW, corresponding to 100,000 infant deaths per year.4,28 Each year, malaria in pregnancy is also responsible for 20% of stillbirths in sub-Saharan Africa.27 Infants born to mothers with placental malaria, particularly infections occurring at the end of pregnancy, have been shown to be at a higher risk of having malaria during the first year of life compared to infants whose mothers had no history of placental infection.13,29 Congenital malaria, defined as the presence of parasites in cord blood or peripheral blood in a newborn in the first 7 days of life in the absence of exposure to infected mosquitoes, has also been described as a consequence of malaria infection in pregnancy, though it is a rare disease in malaria-endemic areas.30,31 Maternal malaria has also been associated with increased risk of infant anemia and other febrile illnesses.5
In low malaria transmission areas, malaria infection in pregnancy has been associated with reductions in birth weight and higher risk of pre-term births in infants.5,19,32 Fetal deaths and congenital malaria have also been reported in these regions.25,31
CONTROL OF MALARIA IN PREGNANCY
In areas of high transmission, the control of malaria in pregnancy is based on three proven interventions: (1) intermittent preventive treatment in pregnancy (IPTp) with sulfadoxine-pyrimethamine (SP) at each scheduled antenatal care (ANC) visit from the second trimester in women of all gravidities, (2) insecticide treated nets (ITNs), and (3) case management (prompt diagnosis and treatment) of malaria cases.33,34 Data from interventional trials and African national datasets have shown that malaria in pregnancy preventive strategies can reduce severe maternal anemia by 40%, low birth weight between 21% and 43%, perinatal mortality by 27% and neonatal mortality between 20% and 60%.6,35,36
Roll Back Malaria’s Global Malaria Action Plan set the target that 100% of pregnant women living in areas of high malaria transmission should receive IPTp.37 Despite an increase in three doses of IPTp coverage from 17% in 2015 to 32% in 2020, coverage remains well below the target, underscoring the substantial number of missed opportunities, given that 57% of women received at least one dose of IPTp in 2020.8 The utilization of community health workers to deliver IPTp has been recently recommended by WHO as an additional delivery method to be explored, in places where inequities in ANC service and reach exist, while ensuring that ANC attendance is maintained.34 In most low-transmission settings, the control of malaria during pregnancy is addressed primarily through prompt case management of women with fever and the use of ITNs.33,38,39,40
WHO guidelines for the treatment of uncomplicated P. falciparum malaria during the first trimester of pregnancy recommend the use of quinine associated with clindamycin for 7 days.41 These recommendations are mainly driven by the fact that quinine and clindamycin are safe and effective. Artemisinins are not currently recommended during the first trimester of pregnancy, despite their appealing attributes,42 due to concerns on the safety of artemisinins during this period.40 For the second and third trimester, the guidelines are the same as for non-pregnant adults; that is to say any artemisinin-based combination treatment (ACT) recommended as first-line treatment (artemether–lumefantrine, artesunate–amodiaquine, artesunate–mefloquine, dihydroartemisinin–piperaquine, or artesunate plus SP) can be used in pregnancy.43 Malaria caused by non-falciparum species should be treated with chloroquine (CQ) in the first trimester (quinine for chloroquine-resistant infections), and with any ACT (except for artesunate plus sulfadoxine-pyrimethamine) in second and third trimester or CQ.41 Severe malaria in pregnancy (any malaria species) is a medical emergency (WHO, 2010).43 Intensive care and prompt parenteral antimalarial treatment are crucial to the mother’s survival.44 Intravenous artesunate is the drug of choice.41
IMMUNOPATHOLOGICAL MECHANISMS BEHIND THE POOR OUTCOMES OBSERVED IN MALARIA IN PREGNANCY AND IMPLICATIONS FOR VACCINE DEVELOPMENT AND EVALUATION
Two mechanisms may explain susceptibility to P. falciparum malaria infections in pregnant women (see Figure 1). First, the infecting parasites express a surface protein (VAR2CSA) that binds to a distinct receptor in the placenta. VAR2CSA belongs to the family of proteins called P. falciparum erythrocyte membrane protein 1 (PfEMP1),45 a family of large and highly polymorphic proteins, encoded by about 60 var genes per haploid genome. These proteins are expressed on the surface of infected erythrocytes and mediate cytoadhesion to several host receptors. In particular, VAR2CSA binds to placental chondroitin sulphate A (CSA) expressed by syncytiotrophoblasts. Therefore, this variant is largely unknown to the immune system before first pregnancy in endemic areas.46 During pregnancy, P. falciparum-infected erythrocytes accumulate in the placenta through adhesion to CSA,47 leading to placental inflammation, the obstruction of the maternofetal exchanges and to severe clinical outcomes for both mother and child. The second potential mechanism of susceptibility involves the immuno-modulation that occurs during pregnancy, driven by factors such as increased plasma cortisol and prolactin, among many others, that inhibit the inflammatory responses necessary for pathogen control.48 The combination of both these factors may contribute to the susceptibility to malaria in pregnant women. See Figure 2 for the relationship between endpoints and components of immunity.
1
The life cycle of Plasmodium falciparum infection in the pregnant woman. Reproduced with permission from ISGlobal / Armand Grand©️.
2
Relationship between endpoints, components of protective immunity, and additional co-factors in malaria infection. (Adapted from Menéndez et al. 2011.49)
At the placental level, the sequestration of infected erythrocytes promotes the infiltration of monocytes and macrophages in the intervillous spaces of the placenta, leading to placental tissue damage.50,51,52 These cells induce significant inflammatory responses via high concentrations of IL-1β, IL-8, TNF-α, and IFN-γ.47,53,54,55 In addition, high concentrations of chemo-attractants factors, such as MCP-1, MIP-1α & β, I-309 and IP-10 are observed.47,55,56,57,58 The placental inflammation promotes placental lesions and exchanges imbalance that may be deleterious for intrauterine growth.59,60 Disruption of angiogenesis through elevated levels of VEGF/Flt1 in the second trimester has also been reported.61 The consequences of this local inflammation are maternal anemia, in utero growth retardation and trans-placental transfer of soluble parasite antigens to the fetus.59,62 Moreover, infected erythrocytes sequestered in the placenta activate monocytes via the expression of CD54 and MHC-II, whereas placental T cells are functionally unable to respond to monocytes63 and to initiate a potent immune response against the parasite. Importantly, several studies have shown that HIV infection can impair P. falciparum-specific antibody responses in pregnant women against a wide range of parasite antigens,64,65 possibly through impairment of memory B-cells mediating long-term humoral immunity,66,67 which increase the risk of malaria associated with HIV infection.15
RATIONALE FOR TESTING MALARIA VACCINES TO PREVENT MALARIA IN PREGNANCY
Malaria during pregnancy continues to be a major cause of maternal and infant morbidity and mortality in endemic areas.7 Important progress has been made in recent years, especially in sub-Saharan Africa, with the introduction of strategies to prevent malaria in pregnancy consisting of intermittent preventive treatment and insecticide-treated nets.7
However, existing tools and policies fail to fully protect the mother and the infant, the two populations most at risk of malaria, from the consequences of the infection in pregnancy. The first important gap in malaria prevention is that most women do not present for antenatal care until mid-gestation. Thus, women and their fetuses are left unprotected during the first half of pregnancy, when parasitemia prevalence may peak and the fetus is developmentally at its most vulnerable. A second gap occurs in the second half of pregnancy; only 32% of pregnant women received at least three doses of IPTp during their pregnancy in 2020, highlighting the missed opportunities given that 74% of pregnant women used ANC services at least once during pregnancy.68 Also, in Africa, at least one million pregnancies are co-infected with malaria and HIV annually. In HIV-infected pregnant women living in areas with high HIV prevalence, universal cotrimoxazole prophylaxis (CTXp) is recommended to prevent opportunistic infections.8 However, IPTp with SP is contraindicated in women on CTXp due to potential adverse effects. Thus, even though IPTp is a life-saving intervention it cannot be administered to the most vulnerable group, HIV-infected women.6,36,69 Thus, paradoxically the most susceptible women to malaria are currently the least protected.70
These challenges in the implementation of preventive strategies for malaria in pregnancy control have fostered efforts to explore other preventive tools that could overcome these problems.7 A vaccine to prevent malaria in pregnancy could potentially be an approach that, combined with the existing strategies, would reduce malaria burden in pregnancy.7 Also, it would be one of the few vaccines that could protect both the mother and the infant. Modeling studies assessing existing tools and vaccines could help to understand whether these efforts would be cost-effective and therefore worth investing.
CONSIDERATIONS FOR CLINICAL EVALUATION OF VACCINES TO PREVENT MALARIA IN PREGNANT WOMEN
WHO Malaria Vaccine Advisory Committee (MALVAC), that provided advice to the Initiative for Vaccine Research (IVR) on strategic priorities, activities and technical issues related to the global effort to develop vaccines to prevent malaria, noted the importance of testing any effective vaccines as soon as possible when available in the target group of pregnant women.71
However, the rationale and approaches for the development and clinical evaluation of malaria vaccines for pregnancy demands specific considerations because of the particular and complex characteristics of the pregnancy status in terms of immunity and safety issues. Also, it raises multiple questions and challenges from basic science and clinical development to clinical impact and vaccine delivery and implementation.7
Effect on malaria-specific immunity and role of co-factors
Major questions include the potential effects of vaccination against malaria in pregnancy on malaria-specific immune responses and the risk of malaria in the infant, and the preservation of the specific immunity acquired during first pregnancies in subsequent pregnancies. Also, a key question is how co-factors, such as the HIV infection or the nutritional status may impair the vaccine immune response,7 or the use of other preventive measures such as ITNs and IPTp, host or parasite genetics, and transmission rates.71
Type of vaccines
The most appropriate and effective type of vaccine for prevention of malaria in pregnancy has also been a focus of discussion.7,71 It has been argued that any vaccine reducing the impact of malaria should rapidly be considered for testing of efficacy in preventing morbidity in the highly vulnerable group of pregnant women.71 A vaccine with lifelong efficacy could be given with other child vaccinations but a vaccine with shorter duration of efficacy (e.g. RTS,S/AS01) would require administration during pre-adolescence (similarly as a vaccine for HPV prevention), providing boosting vaccination dose/s prior to pregnancy.71 The pathogenic mechanisms for the increased risk of malaria in pregnancy is critical for elucidating what the most efficacious vaccine to prevent malaria in pregnancy should look like. This would depend on whether placental infection explain all malaria-related adverse pregnancy outcomes. In this case, a pregnancy-specific malaria vaccine targeting the inhibition of parasites binding to placental receptors (e.g., VAR2CSA-based vaccine candidates) would be an adequate vaccine candidate. However, data available are not conclusive of the fact that placental malaria is responsible for all the adverse effects seen in malaria in pregnancy. This is supported by women found to have peripheral but not placental infection at the end of pregnancy, or that a significant number of women at delivery have antibodies against placental parasites but their placental remains infected.72,73 With this evidence, it may be pondered whether a non-pregnancy-specific malaria vaccine preventing infection (e.g., pre-erythrocytic vaccine targeting sporozoite/liver stage) could be a more appropriate candidate to prevent malaria.
Selection and measurement of endpoints
A major first stage when considering immunization of pregnant women is to decide on the objective of the vaccination, whether this aims to protect the mother, the fetus/infant, or both.7 Depending on the objective, the vaccine could be evaluated to prevent some or all of the malaria-related poor pregnancy outcomes. The endpoints in the assessment of a malaria vaccine in pregnancy will be determined by the decision of what the main outcomes to be prevented should be.7 The potential endpoints that have been proposed to evaluate the efficacy of a vaccine to prevent malaria in pregnancy are summarized in Table 1. The main endpoint for vaccine evaluation, will determine and will be used for sample size calculation, as well as the estimation of the vaccine efficacy (VE).7 The lower the incidence (e.g., if congenital malaria would be the main endpoint) or the lower the contribution of malaria to its occurrence, the bigger the sample size needed to evaluate the VE accurately.74
1
Endpoints to evaluate the efficacy of a vaccine against malaria in pregnancy. (Adapted from Menéndez et al. 2010.7)
Status | |
Maternal | Incidence of peripheral infection Incidence of uncomplication clinical malaria Incidence of complicated (severe) clinical malaria Prevalence of anemia Prevalence of placental infection Incidence of maternal mortality, all-cause and malaria specific |
Fetal/newborn | Incidence of prematurity Incidence of low birth weight Incidence of miscarriage Incidence of stillbirth Prevalence of congenital malaria |
Infant | Incidence of infant morbidity, all-cause and malaria specific Incidence of neonatal mortality Incidence of infant mortality, all-cause and malaria specific |
The measurement of the incidence of maternal peripheral infection would require establishing a system of active detection of infection, which entails substantial investment of resources. This could be done at the ANC visits or through specific surveys in the community.7 The incidence of malaria-related clinical morbidity could be assessed through active and/or passive case detection.7 Placental malaria infection is one of the most important endpoints to measure because it is more specific than peripheral blood examination in detecting the malaria infectious status, and because it can provide information on the past malaria experience of the current pregnancy. Placental infection can be assessed through histological examination of placental biopsies. Parasitological and molecular examination of placental blood is easier to carry out but it is less informative than histology with regard to detection of past and chronic infections. The assessment of fetal endpoints, such as the prevalence of FGR or prematurity requires the evaluation of gestational age in the newborn, since gestational age evaluation through methods, such as last menstrual period or fundal height is frequently unreliable.7 Though not available in most malaria-endemic settings as part of routine ANC, determination of gestational age in the trial through ultrasound assessment is the recommended and most accurate method.75,76,77 For birth weight assessment, the use of digital scales able to discriminate to the nearest gram should be used.78 Demographic surveillance systems need to be in place in selected sites for a precise determination of mortality endpoints, such as maternal, perinatal, and infant mortality. Of particular difficulty in most endemic areas is the assessment of miscarriages.7
If vaccine efficacy is shown against a selected endpoint, for example, a parasitological one such as placental or peripheral infection, it is not straight forward that the results could be extrapolated with certainty to an impact on FGR, prematurity, or maternal anemia. This uncertainty in the extrapolation of the efficacy results to other endpoints depends on the vaccine’s biological target.7 The greater the distance between the vaccine’s biological target (e.g., sporozoite/liver stage) and the trial’s endpoints (e.g., reduction of placental infection), the larger would be the number of co-factors that could interfere with vaccine efficacy on the primary endpoint, and the narrower the applicability of the results (see Figure 1).79
Evaluation of safety
Monitoring and evaluation of safety endpoints is paramount for vaccine trials in pregnant women. Safety endpoints would include reactogenicity, both systemic and local symptoms, and vaccine-related safety endpoints' adverse events (AEs), and serious adverse events (SAEs).78 Pregnancy-specific endpoints require clear definitions for pregnancy outcomes, and maternal and infant complications. Pregnancy outcomes include live birth, non-induced abortion/miscarriage or stillbirth. Numerous pregnancy complications can occur, such as gestational diabetes, gestational hypertension, pre-eclampsia, eclampsia, post-partum hemorrhage, puerperal infection, intrauterine growth restriction, and maternal death, among others. Neonatal complications include preterm birth, neonatal death, low birth weight, neonatal sepsis, and congenital anomalies, among others.77,78
In assessing AEs/SAEs, it is fundamental to understand the background rates of adverse pregnancy outcomes in the trial site, as well as reliable gestational age dating with ultrasound. It is necessary to involve experienced obstetric providers in the conduct of clinical trials in pregnancy and AE/SAE assessment.78 First-trimester exposure is of particular concern for embryotoxicity and teratogenesis, thus reproductive toxicology studies would need to be performed for new investigational malaria vaccine products, or for those already available but not tested with that purpose. Outcomes and AE/SAE should be collected in a standardized fashion, and attention paid to avoid misclassifications, particularly as outcomes may vary by gestational age.78 Recently, the GAIA collaboration (Global Alignment of Immunization Safety Assessments in Pregnant Women) has developed consensus on outcome case definitions for trials during pregnancy.75,76
Timing of vaccination
One of the key issues to elucidate in the evaluation of vaccines to prevent malaria in pregnancy is the timing for the vaccine to be given, thus, who is the target population. For most infections in which maternal immunization is recommended,80,81 the rationale is to prevent infections early in infancy and thus, vaccination is often given early in the third trimester when antibody transfer to the fetus is the greatest. Yet, for malaria in pregnancy, infections early in pregnancy are also harmful for fetal, neonatal, and infant health, and also, malaria has a negative impact on the health of the mother. For these reasons, vaccination to prevent malaria in pregnancy would need to occur prior to childbearing.7 It may be that a malaria vaccine administered to infants alongside the Expanded Program on Immunization (EPI), might induce some long-term immune memory adequate to protect girls later during pregnancy. Leaving this possibility aside, assuming late attendance to ANC continues, that vaccine-specific protective immune responses will require some time to develop, and that the main aim of vaccination is to prevent maternal infection and disease, then it would seem more appropriate to vaccinate girls in the pre-adolescence and before sexual activity begins.7 In this situation, several issues require consideration. Firstly, the need for a long-duration of vaccine protective immunity (not shown yet by any vaccine of those under development or licensed), and the need for re-vaccination (depending of time gap between vaccination and pregnancy) in women of reproductive age. Secondly, whether vaccination should be offered to all pregnancies or just to women in their first pregnancies (primi- and secundigravidae), with the latter being questionable in high transmission areas and in HIV-infected women, in whom the parity-dependent malaria risk pattern vanishes.15
Feasibility of vaccinating the target population
Introducing new vaccines in low-resource settings targeting populations not typically included in the EPI scheme raises questions and challenges that require to be understood beforehand. If a vaccine is given before childbearing, lessons can be learnt from the introduction of the human papillomavirus (HPV) vaccine in pre-adolescent girls in low- and middle-income countries. Demonstration projects were required to understand the most suitable HPV vaccine delivery strategy to maximize vaccine effectiveness; either this was at schools, through outreach activities in the community, mass vaccination campaigns, or at health facilities.82 The acceptability of vaccinating adolescent girls also needs to be carefully assessed before a malaria vaccine trial is planned, including the identification of the barriers to and facilitators for vaccination, as well as its the acceptability among the adolescents themselves but also by their immediate community (e.g., parents, partners, legal tutors) and health workers.7 Also, before a malaria trial is designed in such populations, an important issue to consider includes national policy on the age of consent for adolescents to participate in a research study.
CHARACTETISTICS OF MALARIA VACCINES AND IMMUNOLOGICAL TOOLS CURRENTLY IN CLINICAL DEVELOPMENT OR LICENSED THAT MAY BE CONSIDERED FOR PREVENTION OF MALARIA IN PREGNANCY
The RTS,S/AS01 vaccine (Mosquirix®) is the only malaria vaccine recommended by WHO, for use in children.83 Two vaccine candidates are approaching late-stage clinical evaluation: the R21/MatrixM vaccine targeting PfCSP protein84 and the attenuated whole sporozoite vaccine PfSPZ.85 Additional candidates targeting other malaria life-cycle stages include the Rh5 blood-stage vaccine candidate86 and Pfs25 and Pfs230 vaccine candidates targeting sexual-stage antigens to prevent human-to-mosquito transmission.87 New technologies, such as DNA- and mRNA-based vaccines,88 and delivery platforms such as virus-like particles (VLPs) and vesicle-based technologies, are being explored for use in malaria vaccines.83
To date, no malaria vaccine has ever been tested in pregnant women. Besides, now that RTS,S/AS01 vaccine is available, and recommended for use in children in sub-Saharan Africa, the assessment of its potential role for prevention of malaria in pregnancy would be one expected to be considered.
RTS,S/AS01 (Mosquirix®)
The RTS,S/AS01 vaccine is, so far, the first and only malaria vaccine licensed and recommended by WHO.83 In 2015, RTS,S/AS01 received a positive scientific opinion from the European Medicines Agency.89,90 In 2019, it received national regulatory authorization for use in pilot areas of Ghana, Kenya, and Malawi, where it was provided to children through routine child immunization services as part of pilot phased introduction.83
RTS,S/AS01 is a pre-erythrocytic recombinant protein vaccine, based on the RTS,S recombinant antigen. It comprises the hybrid polypeptide RTS, in which regions of the P. falciparum circumsporozoite protein known to induce humoral and cellular immune responses are covalently bound to the hepatitis B virus surface antigen. The formulation comprises 25 μg of RTS,S with the AS01 adjuvant system.83
RTS,S/AS01 is highly immunogenic, inducing both high titers of circumsporozoite (CS) antibodies and CS-specific CD4 T-cells. No reliable correlate of protection for malaria has been identified.83 Measured anti-CS antibody geometric mean titers (GMTs) are highest 1 month after the third dose; GMTs following the fourth dose are generally lower than this peak level.91 A pivotal phase-3 trial evaluated the efficacy of three or four doses of RTS,S/AS01 in 8922 children aged 5–17 months and 6537 children aged 6–12 weeks at the time of the first dose. There were 11 trial sites across a broad range of transmission settings, including one highly seasonal setting. Among the older children, in the 12 months following administration of the first three doses, vaccine efficacy against clinical malaria (uncomplicated and severe) was 51%, and against severe malaria was 45%.83 At 48 months’ follow-up after the third dose, in children who received a fourth dose, vaccine efficacy against clinical malaria was 39% and against severe malaria 29%.91 A subset of children from the phase-3 trial sites was followed for an additional 3 years (7 years in total) in an open-label extension study.92 Vaccine efficacy remained positive during 7 years of follow-up. Although there was no added protection after the first 48 months of follow up, the impact observed during the first years of protection from the vaccine was not subsequently lost.93 The RTS,S/AS01 vaccine was shown to be safe and well tolerated. There was a small risk (0.25% after the fourth dose) of febrile seizures within 7 days of vaccination, that resolved without long-term consequence.94
Data on the effectiveness of RTS,S were generated through WHO-coordinated evaluation of the pilot phased introductions via the EPI systems in Ghana, Kenya, and Malawi.93 The pilot program showed that RTS,S is feasible to deliver. More than 3 million doses of the vaccine were administered in three African countries and the vaccine had a favorable safety profile. Overall, vaccine introduction increased to over 90% the proportion of children with access to one or more malaria prevention tools (ITN or RTS,S vaccine). More than two-thirds of the children who reportedly did not sleep under an ITN benefited from at least their first dose of RTS,S/AS01. In areas where the vaccine was introduced, there was no decrease in the uptake of other childhood vaccinations, ITN use, or health-seeking behavior for febrile illness.93 RTS,S had a high impact in real-life resulting in a significant reduction (30%) in deadly severe malaria, even in areas with wide use of ITNs and good access to diagnosis and treatment, and 40% reduction in malaria episodes. Modeling studies showed that the vaccine is highly cost effective in areas of moderate to high malaria transmission.93
In October 2021, WHO recommended that the RTS,S/AS01 malaria vaccine should be used for the prevention of P. falciparum malaria in children living in regions with moderate to high malaria transmission, as defined by WHO, and provided in a four-dose schedule in children from 5 months of age.83 RTS,S/AS01 became the first malaria vaccine recommended by WHO for broad use among children in areas with moderate to high transmission of P. falciparum malaria. The recommendation was informed by the results from earlier clinical trials and WHO-coordinated pilot program that had reached more than 800,000 children in three African countries since 2019. The next steps for the broader use of RTS,S as a new preventive tool in the malaria control toolkit include the development and implementation of a framework to guide the allocation of doses of malaria vaccine as supplies of the RTS,S/AS01 vaccine are expected to be limited in the short to medium term, and demand is expected to be high.83
In the assessment of the role of the RTS,S/AS01 vaccine for immunization against malaria in pregnancy, the potential benefits and the favorable characteristics of the vaccine, at the same time that limitations in the type of immune response generated and the potential hurdles in implementation need to be considered. RTS,S/AS01 is the first vaccine licensed and recommended for use in children, and then, in principle, available for potential evaluation in other population groups. Also, the significant amount of evidence generated during the clinical development of the RTS,S/AS01 shows that it is safe and highly immunogenic in children. That being said, one of the critical issues of this vaccine is the duration of the protection; 12 months after three doses, the level of efficacy observed was 51%, and the need for a booster dose to extend protection beyond that period was required (vaccine efficacy against clinical malaria 39% at 48 months' follow up). This seems far from the ideal immunological profile for a vaccine to be administered before childbearing age to prevent malaria in pregnancy. Another important aspect would be the real availability of doses of the vaccine, firstly for clinical evaluation, and eventually for effective implementation in pregnant women given that supplies, at least on the short–mid term, of the RTS,S/AS01 are limited and demand high for its use in children.
PfSPZ vaccine candidate
There are dozens of malaria vaccine candidates under development at present. Of these, PfSPZ vaccine developed by Sanaria, Inc. has the requisite evidence for safety and efficacy to be considered for testing in pregnant women. Pf sporozoites (PfSPZ) are produced in aseptically raised Anopheles stephensi mosquitoes fed on lab-cultured Pf gametocytes, which develop into sporozoites in infected mosquitoes and localize to salivary glands. Radiation-attenuated PfSPZ are prepared by irradiating mosquitoes at this parasite stage, and salivary glands are dissected to release sporozoites. PfSPZ undergo purification and cryopreservation in liquid nitrogen vapor phase (LNVP), all in compliance with current good manufacturing practices (CGMPs) and regulatory requirements. The irradiated product is referred to as "PfSPZ Vaccine".95,96
PfSPZ vaccine has multiple attributes that contribute to its safety profile. The vaccine comprises the non-pathogenic (“clinically silent”) sporozoite and liver stages of Pf. Irradiated PfSPZ are metabolically active and invade hepatocytes but cannot multiply beyond this stage of Pf development thereby avoiding the blood stage of parasite development that causes disease and death. No adjuvant is included in the PfSPZ vaccine preparations tested thus far.
Multiple trials of PfSPZ vaccine have been completed97 with reassuring safety results at different doses and schedules. These trials include six trials in Europe and the US as well as 13 in malaria-exposed adults, children, and infants in Africa. Clinical trials have assessed as few as two doses and as many as six doses. Numerous regimens include widely spaced (for example, weeks 1, 13, 19),98 condensed [days 1, 8, 29 (ClinicalTrials.gov ID NCT03521973)], and complex regimens (multi-dose prime with late boost).99,100 Ten different dose strengths have been tested, from 7.5 × 103 to 2.7 × 106 PfSPZ per intravenous injection. The vaccine has a remarkable safety record with an AE profile similar to normal saline in reports to date. Sanaria vaccine developers have down-selected a regimen of 9 × 105 PfSPZ administered on days 1, 8, and 29 to advance to phase 3 testing.
In collaboration with Sanaria, Inc., scientists at the Malaria Research and Training Center (MRTC) in Bamako, Mali and the Laboratory of Malaria Immunology and Vaccinology (LMIV) at U.S. National Institutes of Health have initiated a clinical development program that will assess PfSPZ vaccine regimens for their safety and efficacy to prevent malaria during pregnancy. Following trials that showed the safety and efficacy of PfSPZ vaccine as a five-dose101 and three-dose regimen in Malian adults,98 MRTC and LMIV scientists have undertaken trials (ClinicalTrials.gov IDs NCT03510481 and NCT03989102) that assess 1-month PfSPZ vaccine regimens in young Malian adults without or with presumptive antimalarial treatments given before vaccine administration. An accelerated vaccine schedule acknowledges the need to induce protection as early in gestation as possible, and any requirement for presumptive antimalarial treatment before vaccine administration will need to be integrated into the existing IPTp program for pregnant women.
As part of this clinical development program, the teams are conducting a phase 2, randomized, double blind, placebo-controlled study to assess PfSPZ vaccine administered before conception as a strategy to prevent malaria during pregnancy. This phase 2 trial is examining 9 × 105 PfSPZ (n = 100) or 1.8 × 106 PfSPZ (n = 100), or placebo (normal saline; n = 100) in healthy 18–38-year-old non-pregnant women who, at enrolment, anticipated becoming pregnant in the near future. Women received vaccine on study days 1, 8, and 29, and have been actively followed post-vaccination for pregnancy. Women who became pregnant during study year 1 were followed through delivery, and then for 1 year post-partum alongside their infants. The study was extended for a second malaria season (September 2020 to February 2021), without any additional study intervention, to explore durability of vaccine efficacy. Pregnancies during study year 2 are being followed to delivery for maternal–newborn outcomes.
Based on progress in clinical development and the strong safety profile, MRTC and LMIV researchers plan trials with Sanaria, Inc. that will administer PfSPZ vaccine during pregnancy,78 and the proposed trials demonstrate one approach to maternal immunization studies with a malaria vaccine, pending review and approval by regulatory authorities. An initial phase 1 safety trial will examine the safety of PfSPZ vaccine administered after the first trimester. The proposed design envisions a randomized, double-blind, placebo-controlled study of a 1-, 8-, 29-day regimen of 9 x 105 PfSPZ of PfSPZ vaccine or placebo (normal saline) in healthy pregnant women and their fetus/newborn. The plan involves two cohorts (third-trimester and second-trimester cohorts) with two arms (PfSPZ vaccine, normal saline placebo) and associated offspring. PfSPZ vaccine will be administered at time points after women receive routine IPTp doses, in order for the vaccine immune response to benefit from blood-stage parasite clearance/suppression. Third-trimester women will be enrolled first, will receive all three doses of vaccine, and will undergo safety reviews before further enrolments. Afterward, second-trimester women will be enrolled and vaccinated. First immunizations will be administered to women between 16 0/7 to 32 6/7 weeks of gestation, and immunizations will be completed prior to term delivery. Both offspring and post-partum women will be followed for 12 months post-delivery.
Phase 1 safety trial results will be reviewed by the study team and regulatory oversight bodies to determine whether to advance to efficacy testing in pregnant women. An initial phase 2 study could vaccinate second-/third-trimester women and assess efficacy as a reduction in the risk of blood stage infections during the remainder of gestation including delivery. Subsequent to phase 2 testing in second-/third-trimester women, strategies to test PfSPZ vaccine in first-trimester women can be developed.
Ultimately, women might be offered a menu of PfSPZ vaccine options to consider alongside anti-vector measures and chemoprevention. Seasonal vaccination before conception could protect women who become pregnant during the malaria season. Booster doses could then be offered early in pregnancy for women who had received a primary vaccine series before conception. Alternatively, a primary vaccine series could be offered as early in pregnancy as possible for unvaccinated women. Achieving protective immunity as early as possible during pregnancy will offer maximum benefits to pregnant women and their offspring.
VAR2CSA-based vaccine candidates
The prevalence of placental malaria sharply decreases with successive pregnancies.5 This protection is associated with the development of antibodies directed towards the surface of P. falciparum-infected erythrocytes from placental origin that express var2csa.102 This observation has supported the use of VAR2CSA as a leading candidate for a vaccine aiming to block parasite sequestration in the placenta. After years of preclinical characterization of potential VAR2CSA-based vaccine candidates, two subunits from the N-terminal fragment of VAR2CSA, which encompass the minimal CSA-binding motifs,103 have entered clinical testing in both malaria-naïve and P. falciparum-exposed non-pregnant women in two distinct phase I clinical trials (ClinicalTrials.gov, NCT02658253 and NCT02647489). Both clinical trials were designed as randomized, double-blind, placebo-controlled, dose escalation trials with the objective of evaluating the safety and immunogenicity of three intramuscular vaccinations with progressively higher doses. PAMVAC originated from the FCR3 variant of VAR2CSA spanning the ID1-DBL2X-ID2a fragment, produced in a Drosophila S2 cell system,104 adjuvanted with Alhydrogel® and induced adhesion-blocking antibodies with strain transcending activity in rodents.105 PRIMVAC vaccine was designed from the DBL1-ID1-DBL2X fragment of 3D7 variant, expressed in an E. coli SHuffle® cell system, adjuvanted with Alhydrogel® or Glucopyranosyl Lipid A Adjuvant-Stable Emulsion (GLA-SE), and induced antibodies in rats with CSA binding inhibition against parasites expressing different variants of VAR2CSA.106,107,108 Participants in phase I trials received three immunizations at 4-week intervals, and both vaccines have appeared to be safe and well-tolerated by the volunteers.104,108 Both PAMVAC and PRIMVAC-induced vaccine-specific antibodies with levels increasing after each vaccination and reaching the highest level after the third vaccination.104,108 However, antibody levels gradually decreased for both vaccines. IgG1 and IgG3 emerged as the major subclasses of antibodies induced by VAR2CSA-based vaccines,108 and both subclasses efficiently interact with most Fc receptors on immune effector cells.
The preclinical and phase I clinical trial results for PAMVAC and PRIMVAC are highly encouraging and confirm the feasibility of developing a placental malaria vaccine through further clinical testing. However, evidence is still lacking about the efficacy of these vaccines to reduce the adverse consequences of malaria in pregnancy. A recent systematic review and meta-analysis could not identify evidence that antibody response towards a specific VAR2CSA antigen is associated with protection from placental malaria.109 The capacity of anti-VAR2CSA antibodies to block the adhesion of CSA-binding infected erythrocytes is thought to play a major role in protection72 but accumulating evidence suggests that other antibody-dependent effector mechanisms, such as opsonic phagocytosis,110,111,112 could also actively participate in parasite clearance. Further information about the longevity of the immune response in vaccinated women and its development upon natural infections may guide large-scale phase II clinical trials. Similarly, novel approaches to increase vaccine immunogenicity and the production of cross-reactive antibodies against different VAR2CSA variants are required. Moreover, the harmonization of clinical trial procedures and the standardization of immunoassays113 can allow the comparability of results with the different vaccines.
mRNA-based vaccine platform
Vaccines based on messenger RNA (mRNA) have been proven successful in protecting populations against Coronavirus Disease 19 (COVID-19) and its most severe consequences. The mRNA technology provide a promising new platform that generates hope for a whole new generation of vaccines that could protect people from devastating infectious diseases from HIV/AIDS to malaria.114 An advantage of existing mRNA technology over traditional approaches in vaccine development is the rapid transition from target discovery to manufacture and testing at an unprecedented pace.114 Also, the versatility to deliver complex, multi-antigens vaccines by combining targets and broad immunity, and potentially adjust for variants and mutations. Formulations are fairly stable and fieldable under conventional conditions, and more cost-effective compared with recombinant protein technologies. However, mRNA vaccines in low- and middle-income countries, where malaria burden concentrates, will certainly need to address major issues such as cold chain and storage capabilities challenges, which may be mitigated if technological transfer occurs to these areas and vaccine production is locally established.
BioNTech, which developed a COVID-19 vaccine in partnership with Pfizer, aims to develop the first mRNA malaria vaccine and plans to begin clinical trials by the end of 2022.114,115,116 Other biopharmaceutical companies (e.g., GlaxoSmithKline) and small biotechs (e.g., CureVac) are investing in the technology, aided by funding from governments and private foundations, and working in new mRNA vaccines, including also malaria.114 Recently, scientists from Walter Reed Army Institute of Research and Naval Medical Research Center, developed a novel vaccine candidate based on mRNA technology that protects against malaria in animal models.117 They selected the immunodominant coat protein of the invasive stage of the malaria parasite, circumsporozoite protein (PfCSP), as the target to evaluate for the protective potential of mRNA malaria vaccines in mice, and showed protective potential of a PfCSP mRNA-lipid nanoparticle (LNP) against, lethal, rodent-malaria transgenic parasites.117
A mRNA-based malaria vaccine may help in the global goal of malaria elimination. But infectious diseases have multiple and challenging mechanisms, particularly malaria due to the complexity of the parasite that causes the disease, and the immune system is notoriously intricate. This may translate that the mRNA technology might not work in diseases other than COVID-19 if their antigens produce less robust immune responses.114 Also, the type of protection (preventing infection vs. severe disease), duration of the response, and other characteristics of immune parameters remain to be seen. However, the accumulatively proven safety profile of mRNA-based COVID-19 vaccines in pregnant women and the robust immune responses elicited (comparable to non-pregnant populations), makes evaluation of mRNA-based malaria vaccines, should a safety and efficacy of a vaccine candidate be demonstrated, a priori appropriate for such a special group, at least worth exploring.
Monoclonal antibodies for malaria
Aside from their application in vaccine design, monoclonal antibodies (mAb) are a promising solution to the problem of overcoming host and parasite factors that can pose challenges to the generation of highly protective and durable vaccine-induced immunity to malaria.118,119 Improvements in production and manufacturing have reduced cost barriers to the prophylactic use of mAbs. Furthermore, as opposed to small molecule development, antibodies may be less prone to off-target safety and toxicity issues and, thus, may offer advantages when deployed in vulnerable populations such as pregnant women or immunocompromised individuals.118
In a recent phase 2 trial, a single infusion of the antimalarial monoclonal antibody CIS43LS had no evident safety concerns and protected healthly adults against P. falciparum infection during a 6-month malaria season in Mali.120 In addition, an investigational monoclonal antibody called L9LS protected most recipients against malaria after controlled infection in a small phase 1 clinical trial.119 Deemed a next-generation monoclonal antibody, L9LS performed substantially better than previous antimalarial antibodies in preclinical studies.121 First, it targets a more tightly defined region of the CSP-1 protein, with high affinity, which makes it three times more potent than previous anti-CSP-1 antibodies.122 Second, L9LS antibody harbors a key design feature that increases neonatal Fc receptor binding, which increases its half-life in the blood.122 This last trial provided a proof of principle that prevention of malaria can be achieved with a next-generation monoclonal antibody.119 Two phase 2 clinical trials are ongoing in Kenya (ClinicalTrials.gov number, NCT05400655) and Mali (NCT05304611) to assess protection against perennial and seasonal transmission using a small subcutaneous administration of L9LS in young children.
Currently, no antimalaria antibody is approved for clinical use. If successfully developed, adding antibodies to chemoprevention and vaccine therapies might substantially reduce malaria-related illness and deaths.121 The subcutaneous injection, taken once a season, could make antibodies cost-effective for treating high-risk groups including infants, young children, and pregnant women in regions where malaria is endemic.121 Given that monoclonal antibodies do not contain adjuvants and are not intended to stimulate an immune response, the ability to provide several months of protection with administration of a single dose would be valuable for prevention of malaria in pregnancy.119 Finally, as with vaccines, the use of mAbs as an intervention would need to demonstrate safety and duration of protection and consider factors related to manufacturing capacity, formulation, cost of goods, route of administration and programmatic suitability.118
CONCLUSIONS
Malaria in pregnancy continues to be a foremost health problem for mothers and infants in endemic areas. Successful control of malaria in pregnancy can save lives of mothers and babies and is a high global health priority.123 However, existing preventive tools are failing to entirely protect pregnant women and their infants from the consequences of malaria infection in pregnancy due to challenges in implementation. This situation has stimulated the need to explore other preventive strategies that could overcome these problems.
Immunization is a proven success story for global health and development, saving millions of lives every year. A vaccine to prevent malaria in pregnancy would be an ideal strategy to effectively protect mother and infants from the harmful consequences of the infection, which combined with current tools would maximize protection. However, the rationale and approach for the clinical development and evaluation of malaria vaccines for pregnancy demands specific considerations because of the complex characteristics and challenges of the pregnancy status in terms of immunity and safety issues.
Several candidate products are presently in the clinical development and evaluation pathway as potential tools to prevent malaria in pregnancy, such as VAR2CSA-based and PfSPZ vaccine candidates. The first approved malaria vaccine, recommended by the WHO (Mosquirix®) in 2021 for broad use in children is now available and its potential role to prevent malaria in pregnancy might be considered. The mRNA technology, demonstrated to be successful in protecting populations against COVID-19, also offers a promising platform for a new generation of vaccines, including malaria. Finally, monoclonal antibodies for malaria are a promising solution to the problem of overcoming the challenges to the generation of highly protective and durable vaccine-induced immunity in high-risk groups including pregnant women.
Substantial progress has been made thus far in the clinical development and testing of malaria vaccine candidates to prevent malaria in pregnancy. However, challenges remain regarding the adequacy of the current vaccine products and candidates available, such as the type of immune response elicited, the level of efficacy achieved, the duration of protection, or the need for a safety profile proven in the target population.
A fundamental step in developing and testing vaccines against malaria in pregnancy requires the description and consensus of the ideal characteristics of the vaccines and the ways in which such vaccines would be used, the target product profile. Finally, a thorough understanding of the programmatic and implementation issues, specifically which would be the best and most suitable strategy for vaccination of women in malaria endemic countries, is of utmost importance for successful immunization.
PRACTICE RECOMMENDATIONS
Epidemiology of malaria in pregnancy and consequences on maternal and infant health
- Malaria is the most important parasitic infection of humans. Globally, in 2020 there were an estimated 241 million malaria cases in malaria endemic countries and 627,000 malaria deaths.
- P. falciparum is responsible for most deaths and morbidity, and its greatest burden concentrates in sub-Saharan Africa.
- In 2020, in moderate to high transmission countries in WHO Africa region, there were an estimated 33.8 million pregnancies, of which 11.6 million were exposed to malaria infection.
- Pregnant women have a higher risk of malaria infection, greater parasitemia density, and clinical/symptomatic malaria compared to non-pregnant women.
- Malaria susceptibility in pregnancy is modulated by maternal age, parity, gestational age, the level of malaria-specific acquired immunity, and HIV infection.
- Anemia is the most common outcome associated to P. falciparum malaria infection, and a risk factor for maternal death and poor infant outcomes.
- P. falciparum malaria has been estimated to account for 10–20% of maternal mortality in endemic countries.
- Malaria in pregnancy is associated with increased risk of fetal loss (miscarriage and stillbirth), low birth weight, preterm delivery, and neonatal mortality.
Immunopathological mechanisms behind the poor outcomes observed in malaria in pregnancy
The mechanisms behind the susceptibility to P. falciparum malaria in pregnant women include the following:
- Infecting parasites that express a surface protein (VAR2CSA) that binds to a distinct receptor in the placenta. Infected erythrocytes accumulate in the placenta through adhesion to CSA, leading to placental inflammation, obstruction of the maternofetal exchanges and severe clinical outcomes for both mother and child.
- Immuno-modulation that occurs during pregnancy, driven by factors such as increased plasma cortisol and prolactin, among many others, that inhibit the inflammatory responses necessary for pathogen control.
Strategies for control of malaria in pregnancy
In areas of high transmission, the control of malaria in pregnancy is based on three proven interventions: IPTp with SP at each scheduled ANC visit from the second trimester in women of all gravidities, ITNs, and prompt diagnosis and treatment of malaria cases.
Rationale and considerations for evaluation of vaccines to prevent malaria in pregnancy
- Malaria in pregnancy continues to be a foremost health problem for mothers and infants in endemic areas.
- However, existing preventive tools are failing to entirely protect them due to challenges in implementation.
- This situation has stimulated the need to explore other preventive tools such as vaccines.
- Nonetheless, the evaluation and development of vaccines to prevent malaria in pregnancy raises multiple questions and challenges such as the following:
- the effect of vaccination on malaria-specific immune responses and the risk of malaria in the infant, and the role of co-factors as HIV infection or malnutrition;
- what is the most appropriate type of vaccine;
- the selection and measurement of trial endpoints;
- monitoring and evaluation of safety in vaccine trials;
- the timing for the vaccine to be given and who is the target population;
- the feasibility of vaccinating the target population.
Vaccines and immuniological tools for potential consideration for prevention of malaria in pregnancy
- To date, no malaria vaccine has ever been tested in pregnant women.
- Several candidate products are currently in the clinical development pathway, such as the VAR2CSA-based and the attenuated whole sporozoite PfSPZ vaccine candidates to elucidate their potential role to prevent malaria in pregnancy.
- The RTS,S/AS01 vaccine (Mosquirix®) is the only malaria vaccine recommended by WHO for use in children in sub-Saharan Africa. The assessment of its potential role for prevention of malaria in pregnancy may be one expected to be considered.
- New technologies, such as DNA- and mRNA-based vaccines, and delivery platforms such as virus-like particles (VLPs) and vesicle-based technologies, and monoclonal antibodies, are being explored for use in malaria prevention in pregnancy, and offer a promising platform for a new generation of vaccines and immunological products.
Final remarks
- Immunization is a proven success story for global health and development, saving millions of lives every year.
- A vaccine to prevent malaria in pregnancy would be an ideal strategy that, combined with existing tools, would reduce malaria burden in pregnancy.
- Progress has been made thus far in the clinical development of vaccines to prevent malaria in pregnancy, however challenges remain about the immune response elicited, the level of efficacy, the duration of protection, or the need for a proven safety profile in the target population.
- A necessary step on clinical development of vaccines to prevent malaria in pregnancy is the description and consensus of the ideal characteristics of the vaccine; the target product profile.
- A thorough understanding of the programmatic and implementation issues, particularly which would be the best and most suitable strategy for vaccine delivery, is of utmost importance for successful immunization.
CONFLICTS OF INTEREST
The author(s) of this chapter declare that they have no interests that conflict with the contents of the chapter.
Feedback
Publishers’ note: We are constantly trying to update and enhance chapters in this Series. So if you have any constructive comments about this chapter please provide them to us by selecting the "Your Feedback" link in the left-hand column.
REFERENCES
Dellicour S, Tatem AJ, Guerra CA, et al. Quantifying the number of pregnancies at risk of malaria in 2007: a demographic study. PLoS Med 2010;7(1):e1000221. | |
Castillo P, Menéndez C, Mayor A, et al. Massive Plasmodium falciparum visceral sequestration: a cause of maternal death in Africa. England: © 2012 The Authors Clinical Microbiology and Infection © 2012 European Society of Clinical Microbiology and Infectious Diseases. Clin Microbiol Infect 2013;19:1035–41. | |
Mayor A, Rovira-Vallbona E, Machevo S, et al. Parity and placental infection affect antibody responses against Plasmodium falciparum during pregnancy. Infect Immun 2011;79(4):1654–9. | |
Desai M, ter Kuile FO, Nosten F, et al. Epidemiology and burden of malaria in pregnancy. Lancet Infect Dis United States, 2007;7:93–104. | |
Rogerson SJ, Desai M, Mayor A, et al. Burden, pathology, and costs of malaria in pregnancy: new developments for an old problem. Lancet Infect Dis United States: © 2018 Elsevier Ltd, 2018;18:e107 e18. | |
Menéndez C, Bardají A, Sigauque B, et al. Malaria prevention with IPTp during pregnancy reduces neonatal mortality. PLoS ONE 2010;5(2). | |
Menendez C, Alonso P, Universitat de B. Guidelines and considerations for testing malaria vaccines in pregnant women. Hum Vaccin United States, 2010;6:21–6. | |
World malaria report 2021. Geneva: World Health Organization, 2021. Licence: CC BY-NC-SA 3.0 IGO. | |
Duffy PE, Desowitz RS. Pregnancy malaria throughout history: dangerous labors. In: Duffy E, Fried M. (eds.) Malaria in pregnancy: deadly parasite, susceptible host. New York: Taylor & Francis, 2001:1–25. | |
Blacklock DB, Gordon RM. Malaria infection as it occurs in late pregnancy, its relationship to labour and early infancy. Ann Trop Med Parasitol 1925;19:327–65. | |
Wickramasuriya GAW. Some observations on malaria occurring in association with pregnancy. J Obstet Gynaecol Br Empire 1935;42:816–34. | |
Umbers AJ, Aitken EH, Rogerson SJ. Malaria in pregnancy: small babies, big problem. Trends Parasitol England: © 2011 Elsevier Ltd, 2011;27:168–75. | |
Bardají A, Sigauque B, Bruni L, et al. Clinical malaria in African pregnant women. Malaria Journal 2008;7. | |
Fried M, Duffy PE. Malaria during Pregnancy. Cold Spring Harb Perspect Med 2017;7(6). | |
ter Kuile FO, Parise ME, Verhoeff FH, et al. The burden of co-infection with human immunodeficiency virus type 1 and malaria in pregnant women in sub-Saharan Africa. Am J Trop Med Hyg 2004;71(2 Suppl):41–54. | |
UNAIDS Data 2021. Geneva: Joint United Nations Programme on HIV/AIDS, 2021. Licence: CC BY-NC-SA 3.0 IGO. | |
UNAIDS/WHO. Global report: UNAIDS report on the global AIDS Epidemic 2013. WHO Library Cataloguing-in-Publication Data 2013, UNAIDS(JC2502/1/E). | |
González R, Naniche D. HIV and Malaria Interaction in the Pregnant Women. In: Hommel M, Kremsner PG. (eds.) Encyclopedia of Malaria. New York, NY: Springer New York, 2014:1–9. | |
Bardají A, Martínez-Espinosa FE, Arévalo-Herrera M, et al. Burden and impact of Plasmodium vivax in pregnancy: A multi-centre prospective observational study. PLoS Neglected Tropical Diseases 2017;11(6). | |
Marín-Menéndez A, Bardají A, Martínez-Espinosa FE, et al. Rosetting in Plasmodium vivax: A Cytoadhesion Phenotype Associated with Anaemia. PLoS Neglected Tropical Diseases 2013;7(4). | |
Guyatt HL, Snow RW. The epidemiology and burden of Plasmodium falciparum-related anemia among pregnant women in sub-Saharan Africa. Am J Trop Med Hyg 2001;64(1–2 Suppl):36–44. | |
Brabin B, Verhoeff F, et al. The contribution of malaria. Maternal morbidity and mortality. London, UK: RCOG Press, 2002:65–78. | |
Menéndez C, Romagosa C, Ismail MR, et al. An autopsy study of maternal mortality in Mozambique: The contribution of infectious diseases. PLoS Medicine 2008;5(2):0220–6. | |
Poespoprodjo JR, Fobia W, Kenangalem E, et al. Adverse pregnancy outcomes in an area where multidrug-resistant plasmodium vivax and Plasmodium falciparum infections are endemic. Clin Infect Dis 2008;46(9):1374–81. | |
Rijken MJ, McGready R, Boel ME, et al. Malaria in pregnancy in the Asia-Pacific region. Lancet Infect Dis United States: © 2012 Elsevier Ltd, 2012;12:75–88. | |
Moore KA, Simpson JA, Scoullar MJL, et al. Quantification of the association between malaria in pregnancy and stillbirth: a systematic review and meta-analysis. Lancet Glob Health England: © 2017 The Author(s). Published by Elsevier Ltd. This is an Open Access article under the CC BY 4.0 license. Published by Elsevier Ltd, 2017;5:e1101–e12. | |
Saito M, Briand V, Min AM, et al. Deleterious effects of malaria in pregnancy on the developing fetus: a review on prevention and treatment with antimalarial drugs. Lancet Child Adolesc Health England: © 2020 Elsevier Ltd, 2020;4:761–74. | |
Steketee RW, Nahlen BL, Parise ME, et al. The burden of malaria in pregnancy in malaria-endemic areas. Am J Trop Med Hyg 2001;64(1–2 Suppl):28–35. | |
Le Port A, Watier L, Cottrell G, et al. Infections in infants during the first 12 months of life: role of placental malaria and environmental factors. PLoS One 2011;6(11):e27516. | |
Menendez C, Mayor A. Congenital malaria: the least known consequence of malaria in pregnancy. Semin Fetal Neonatal Med Netherlands, 2007;12:207–13. | |
Castellanos ME, Bardají A, Menegon M, et al. Plasmodium vivax congenital malaria in an area of very low endemicity in Guatemala: Implications for clinical and epidemiological surveillance in a malaria elimination context. Malaria Journal 2012;11. | |
Machado Filho AC, da Costa EP, Reis IS, et al. Effects of vivax malaria acquired before 20 weeks of pregnancy on subsequent changes in fetal growth. Am J Trop Med Hyg 2014;90(2):371–6. | |
Hamel MJ, D’Alessandro U. Control of Malaria During Pregnancy: Preventive Strategies. Intermittent Preventive Treatment and Insecticide-Treated Nets. In: Hommel M, Kremsner PG. (eds.) Encyclopedia of Malaria. New York, NY: Springer New York, 2014:1–10. | |
WHO Guidelines for malaria, 3 June 2022. Geneva: World Health Organization, 2022 (WHO/UCN/GMP/2022.01 Rev.2). License: CC BY-NC-SA 3.0 IGO. | |
Garner P, Gulmezoglu AM. Drugs for preventing malaria in pregnant women. The Cochrane Database of Systematic Reviews 2006(4):CD000169. | |
Eisele TP, Larsen DA, Anglewicz PA, et al. Malaria prevention in pregnancy, birthweight, and neonatal mortality: a meta-analysis of 32 national cross-sectional datasets in Africa. Lancet Infect Dis United States: © 2012 Elsevier Ltd, 2012;12:942–9. | |
Partnership RBM. The Global Malaria Action Plan – For a Malaria-Free World. WHO, 2008. | |
Mayor A, Meshnick SR. Diagnostic Tools of Malaria During Pregnancy. In: Hommel M, Kremsner PG. (eds.) Encyclopedia of Malaria. New York, NY: Springer New York, 2014:1–12. | |
WHO. Lives at Risk: Malaria in Pregnancy 2003 [Available from: http://www.who.int/features/2003/04b/en/]. | |
D’Alessandro U, Hamel MJ. Control of Malaria During Pregnancy: Treatment of Uncomplicated Malaria and Complicated Malaria. In: Hommel M, Kremsner PG. (eds.) Encyclopedia of Malaria. New York, NY: Springer New York, 2014:1–18. | |
WHO. Guidelines for the treatment of malaria, 3rd edn. Geneva: World Health Organization, 2015. | |
Saito M, Mansoor R, Kennon K, et al. Efficacy and tolerability of artemisinin-based and quinine-based treatments for uncomplicated falciparum malaria in pregnancy: a systematic review and individual patient data meta-analysis. Lancet Infect Dis 2020;20(8):943–52. | |
D'Alessandro U, Hill J, Tarning J, et al. Treatment of uncomplicated and severe malaria during pregnancy. Lancet Infect Dis 2018;18(4):e133–e46. | |
Nosten F, McGready R, Mutabingwa T. Case management of malaria in pregnancy. Lancet Infect Dis United States, 2007;7:118–25. | |
Baruch DI, Pasloske BL, Singh HB, et al. Cloning the P. falciparum gene encoding PfEMP1, a malarial variant antigen and adherence receptor on the surface of parasitized human erythrocytes. Cell 1995;82(1):77–87. | |
Beeson JG, Reeder JC, Rogerson SJ, et al. Parasite adhesion and immune evasion in placental malaria. Trends Parasitol 2001;17(7):331–7. | |
Rogerson SJ, Hviid L, Duffy PE, et al. Malaria in pregnancy: pathogenesis and immunity. Lancet Infect Dis 2007;7(2):105–17. | |
Bouyou-Akotet MK, Adegnika AA, Agnandji ST, et al. Cortisol and susceptibility to malaria during pregnancy. MICROBES INFECT 2005;7(11–12):1217–23. | |
Clara Menéndez, Vasee S Moorthy, Zarifah Reed, Azucena Bardají, Pedro Alonso & Graham V Brown (2011) Development of vaccines to prevent malaria in pregnant women: WHO MALVAC meeting report, Expert Review of Vaccines, 10:9, 1271-1280, DOI: 10.1586/erv.11.95 | |
Menendez C, Ordi J, Ismail MR, et al. The impact of placental malaria on gestational age and birth weight. J Infect Dis 2000;181(5):1740–5. | |
Ordi J, Ismail MR, Ventura PJ, et al. Massive chronic intervillositis of the placenta associated with malaria infection. Am J Surg Pathol 1998;22(8):1006–11. | |
Rogerson SJ, Pollina E, Getachew A, et al. Placental monocyte infiltrates in response to Plasmodium falciparum malaria infection and their association with adverse pregnancy outcomes. Am J Trop Med Hyg 2003;68(1):115–9. | |
Diouf I, Fievet N, Doucoure S, et al. IL-12 producing monocytes and IFN-gamma and TNF-alpha producing T-lymphocytes are increased in placentas infected by Plasmodium falciparum. J Reprod Immunol 2007;74(1–2):152–62. | |
Fried M, Muga RO, Misore AO, et al. Malaria elicits type 1 cytokines in the human placenta: IFN-gamma and TNF-alpha associated with pregnancy outcomes. J Immunol 1998;160(5):2523–30. | |
Moormann AM, Sullivan AD, Rochford RA, et al. Malaria and pregnancy: placental cytokine expression and its relationship to intrauterine growth retardation. J Infect Dis 1999;180(6):1987–93. | |
Abrams ET, Brown H, Chensue SW, et al. Host response to malaria during pregnancy: placental monocyte recruitment is associated with elevated beta chemokine expression. J Immunol 2003;170(5):2759–64. | |
Mens PF, Bojtor EC, Schallig HD. Molecular interactions in the placenta during malaria infection. Eur J Obstet Gynecol Reprod Biol 2010;152(2):126–32. | |
Suguitan AL Jr, Leke RG, Fouda G, et al. Changes in the levels of chemokines and cytokines in the placentas of women with Plasmodium falciparum malaria. J Infect Dis 2003;188(7):1074–82. | |
Boeuf P, Aitken EH, Chandrasiri U, et al. Plasmodium falciparum malaria elicits inflammatory responses that dysregulate placental amino acid transport. PLoS Pathog 2013;9(2):e1003153. | |
Ismail MR, Ordi J, Menendez C, et al. Placental pathology in malaria: a histological, immunohistochemical, and quantitative study. Hum Pathol 2000;31(1):85–93. | |
Wathen KA, Tuutti E, Stenman UH, et al. Maternal serum-soluble vascular endothelial growth factor receptor-1 in early pregnancy ending in preeclampsia or intrauterine growth retardation. J Clin Endocrinol Metab 2006;91(1):180–4. | |
Rogerson SJ, Brown HC, Pollina E, et al. Placental tumor necrosis factor alpha but not gamma interferon is associated with placental malaria and low birth weight in Malawian women. Infect Immun 2003;71(1):267–70. | |
Diouf I, Fievet N, Doucoure S, et al. Monocyte activation and T cell inhibition in Plasmodium falciparum-infected placenta. J Infect Dis 2004;189(12):2235–42. | |
Dembo EG, Mwapasa V, Montgomery J, et al. Impact of human immunodeficiency virus infection in pregnant women on variant-specific immunity to malaria. Clin Vaccine Immunol 2008;15(4):617–21. | |
Naniche D, Serra-Casas E, Bardaji A, et al. Reduction of antimalarial antibodies by HIV infection is associated with increased risk of Plasmodium falciparum cord blood infection. J Infect Dis 2012;205(4):568–77. | |
De Milito A, Nilsson A, Titanji K, et al. Mechanisms of hypergammaglobulinemia and impaired antigen-specific humoral immunity in HIV-1 infection. Blood 2004;103(6):2180–6. | |
Titanji K, De Milito A, Cagigi A, et al. Loss of memory B cells impairs maintenance of long-term serologic memory during HIV-1 infection. Blood 2006;108(5):1580–7. | |
WHO. Consolidated guidelines on the use of antiretroviral drugs for treating and preventing HIV infection Recommendations for a public health approach June 2013, 2013. | |
Sevene E, González R, Menéndez C. Current knowledge and challenges of antimalarial drugs for treatment and prevention in pregnancy. Expert Opin Pharmacother 2010;11(8):1277–93. | |
González R, Sevene E, Jagoe G, et al. A Public Health Paradox: The Women Most Vulnerable to Malaria Are the Least Protected. PLoS Med 2016;13(5):e1002014. | |
Fried M, Nosten F, Brockman A, et al. Development of vaccines to prevent malaria in pregnant women: WHO MALVAC meeting report. Expert review of vaccines, 2011. | |
Fried M, Nosten F, Brockman A, et al. Maternal antibodies block malaria. Nature 1998;395(6705):851–2. | |
Ricke CH, Staalsoe T, Koram K, et al. Plasma antibodies from malaria-exposed pregnant women recognize variant surface antigens on Plasmodium falciparum-infected erythrocytes in a parity-dependent manner and block parasite adhesion to chondroitin sulfate A. J Immunol United States, 2000;165:3309–16. | |
Smith PG, Morrow RH. (eds.) Methods for field trials of interventions against tropical diseases: a "toolbox", Oxford University Press, 1991. | |
Bonhoeffer J, Kochhar S, Hirschfeld S, et al. Global alignment of immunization safety assessment in pregnancy – The GAIA project. Vaccine 2016;34(49):5993–7. | |
Kochhar S, Bauwens J, Bonhoeffer J. Safety assessment of immunization in pregnancy. Vaccine 2017;35(48 Pt A):6469–71. | |
Stuurman AL, Sharan A, Jahagirdar S, et al. WHO global vaccine safety multi-country collaboration project on safety in pregnancy: Assessing the level of diagnostic certainty using standardized case definitions for perinatal and neonatal outcomes and maternal immunization. Vaccine X 2021;9:100123. | |
Healy SA, Fried M, Richie T, et al. Malaria vaccine trials in pregnant women: An imperative without precedent. Vaccine 2019;37(6):763–70. | |
Guinovart C, Alonso PL. Methods for determining vaccine efficacy and effectiveness and the main barriers to developing a fully deployable malaria vaccine. Am J Trop Med Hyg United States, 2007;77:276–81. | |
Fiore AE, Uyeki TM, Broder K, et al. Prevention and control of influenza with vaccines: recommendations of the Advisory Committee on Immunization Practices (ACIP), 2010. MMWR Recomm Rep 2010;59(Rr-8):1–62. | |
Centers for Disease Control and Prevention. Updated Recommendations for Use of Tetanus Toxoid, Reduced Diphtheria Toxoid, and Acellular Pertussis Vaccine (Tdap) in Pregnant Women – Advisory Committee on Immunization Practices (ACIP), 2012. Mmwr 2013;62(07):131–135. | |
Bardaji A, Mindu C, Augusto OJ, et al. Awareness of cervical cancer and willingness to be vaccinated against human papillomavirus in Mozambican adolescent girls. Papillomavirus Research 2018;5:156–62. | |
World Health Organization = Organisation mondiale de la Santé. Malaria vaccine: WHO position paper – March 2022 – Rapport mensuel des cas de dracunculose, Janvier 2022. Weekly Epidemiological Record = Relevé épidémiologique hebdomadaire, 2022;97(09):60–78. Geneva = Genève. https://apps.who.int/iris/handle/10665/352337. | |
Datoo MS, Natama MH, Somé A, et al. Efficacy of a low-dose candidate malaria vaccine, R21 in adjuvant Matrix-M, with seasonal administration to children in Burkina Faso: a randomised controlled trial. Lancet 2021;397(10287):1809–18. | |
Butler D. Promising malaria vaccine to be tested in first large field trial. Nature England, 2019. | |
Minassian AM, Silk SE, Barrett JR, et al. Reduced blood-stage malaria growth and immune correlates in humans following RH5 vaccination. Med (N Y) 2021;2(6):701–19 e19. | |
Safety and immunogenicity of Pfs25M-EPA/AS01 and Pfs230D1M-EPA/AS01 vaccines, transmission blocking vaccines against Plasmodium falciparum, at full and fractional dosing in adults in Mali. National Institutes of Health Clinical Center (https://clinicaltrials.gov/ct2/show/NCT02942277, accessed June 2022 ). | |
Draper SJ, Angov E, Horii T, et al. Recent advances in recombinant protein-based malaria vaccines. Vaccine 2015;33(52):7433–43. | |
Mosquirix: Opinion on medicine for use outside EU. Amsterdam, The Netherlands. European Medicine Agency (https://www.ema.europa.eu/en/opinion-medicine-use-outside-EU/human/mosquirix, accessed June 2022). | |
Assessment report Mosquirix. Amsterdam: European Medicines Agency, 2015 (https://www.ema.europa.eu/en/documents/outside-eu-assessment-report/mosquirix-public-assessment-report_en.pdf, accessed June 2022). | |
Efficacy and safety of RTS,S/AS01 malaria vaccine with or without a booster dose in infants and children in Africa: final results of a phase 3, individually randomised, controlled trial. Lancet 2015;386(9988):31–45. | |
Tinto H, Otieno W, Gesase S, et al. Long-term incidence of severe malaria following RTS,S/AS01 vaccination in children and infants in Africa: an open-label 3-year extension study of a phase 3 randomised controlled trial. Lancet Infect Dis United States: © 2019 Elsevier Ltd, 2019;19:821–32. | |
Full evidence report on the RTS,S/AS01 malaria vaccine. Geneva: World Health Organization (https://cdn.who.int/media/docs/default-source/immunization/mvip/full-evidence-report-on-the-rtss-as01-malaria-vaccine-for-sage-mpag-(sept2021).pdf?sfvrsn=c9737be_5, accessed June 2022). | |
Guerra Mendoza Y, Garric E, Leach A, et al. Safety profile of the RTS,S/AS01 malaria vaccine in infants and children: additional data from a phase III randomized controlled trial in sub-Saharan Africa. Hum Vaccin Immunother 2019;15(10):2386–98. | |
Hoffman SL, Billingsley PF, James E, et al. Development of a metabolically active, non-replicating sporozoite vaccine to prevent Plasmodium falciparum malaria. Human Vaccines 2010;6(1):97–106. | |
Epstein JE, Tewari K, Lyke KE, et al. Live attenuated malaria vaccine designed to protect through hepatic CD8(+) T cell immunity. Science 2011;334(6055):475–80. | |
Duffy PE, Patrick Gorres J. Malaria vaccines since 2000: progress, priorities, products. NPJ Vaccines 2020;5(1):48. | |
Sissoko MS, Healy SA, Katile A, et al. Safety and efficacy of a three-dose regimen of Plasmodium falciparum sporozoite vaccine in adults during an intense malaria transmission season in Mali: a randomised, controlled phase 1 trial. Lancet Infect Dis 2022;22(3):377–89. | |
Jongo SA, Church LWP, Nchama V, et al. Multi-Dose Priming Regimens of PfSPZ Vaccine: Safety and Efficacy against Controlled Human Malaria Infection in Equatoguinean Adults. Am J Trop Med Hyg 2022. | |
Lyke KE, Singer A, Berry AA, et al. Multidose Priming and Delayed Boosting Improve Plasmodium falciparum Sporozoite Vaccine Efficacy Against Heterologous P. falciparum Controlled Human Malaria Infection. Clin Infect Dis 2021;73(7):e2424–e35. | |
Sissoko MS, Healy SA, Katile A, et al. Safety and efficacy of PfSPZ Vaccine against Plasmodium falciparum via direct venous inoculation in healthy malaria-exposed adults in Mali: a randomised, double-blind phase 1 trial. Lancet Infect Dis 2017;17(5):498–509. | |
Ataide R, Mayor A, Rogerson SJ. Malaria, primigravidae, and antibodies: knowledge gained and future perspectives. Trends Parasitol 2014;30(2):85–94. | |
Clausen TM, Christoffersen S, Dahlback M, et al. Structural and functional insight into how the Plasmodium falciparum VAR2CSA protein mediates binding to chondroitin sulfate A in placental malaria. J Biol Chem 2012;287(28):23332–45. | |
Mordmuller B, Sulyok M, Egger-Adam D, et al. First-in-human, Randomized, Double-blind Clinical Trial of Differentially Adjuvanted PAMVAC, A Vaccine Candidate to Prevent Pregnancy-associated Malaria. Clin Infect Dis 2019;69(9):1509–16. | |
Nielsen MA, Resende M, de Jongh WA, et al. The Influence of Sub-Unit Composition and Expression System on the Functional Antibody Response in the Development of a VAR2CSA Based Plasmodium falciparum Placental Malaria Vaccine. PLoS One 2015;10(9):e0135406. | |
Chene A, Gangnard S, Dechavanne C, et al. Down-selection of the VAR2CSA DBL1–2 expressed in E. coli as a lead antigen for placental malaria vaccine development. NPJ Vaccines 2018;3:28. | |
Chene A, Gangnard S, Guadall A, et al. Preclinical immunogenicity and safety of the cGMP-grade placental malaria vaccine PRIMVAC. EBioMedicine 2019;42:145–56. | |
Sirima SB, Richert L, Chene A, et al. PRIMVAC vaccine adjuvanted with Alhydrogel or GLA-SE to prevent placental malaria: a first-in-human, randomised, double-blind, placebo-controlled study. Lancet Infect Dis 2020;20(5):585–97. | |
Cutts JC, Agius PA, Zaw L, et al. Pregnancy-specific malarial immunity and risk of malaria in pregnancy and adverse birth outcomes: a systematic review. BMC Med 2020;18(1):14. | |
Keen J, Serghides L, Ayi K, et al. HIV impairs opsonic phagocytic clearance of pregnancy-associated malaria parasites. PLoS Med 2007;4(5):e181. | |
Ataide R, Mwapasa V, Molyneux ME, et al. Antibodies that induce phagocytosis of malaria infected erythrocytes: effect of HIV infection and correlation with clinical outcomes. PLoS One 2011;6(7):e22491. | |
Jaworowski A, Fernandes LA, Yosaatmadja F, et al. Relationship between human immunodeficiency virus type 1 coinfection, anemia, and levels and function of antibodies to variant surface antigens in pregnancy-associated malaria. Clin Vaccine Immunol 2009;16(3):312–9. | |
Chene A, Houard S, Nielsen MA, et al. Clinical development of placental malaria vaccines and immunoassays harmonization: a workshop report. Malar J 2016;15:476. | |
mRNA vaccines provide a promising new weapon. Neil Savage. Nature 2021;598. | |
BioNTech to develop mRNA malaria vaccine; unveils ambitions for Africa vaccine supply. By Rachel Arthur. BioPharma-Reporter.com, 2021. | |
WHO Director-General´s opening remarks at the mRNA Malaria Vaccine event. WHO, 2021. | |
Mallory KL, Taylor JA, Zou X, et al. Messenger RNA expressing PfCSP induces functional, protective immune responses against malaria in mice. NPJ Vaccines 2021;6(1):84. | |
Malaria vaccines: preferred product characteristics and clinical development considerations. Geneva: World Health Organization; 2022. Licence: CC BY-NC-SA 3.0 IGO. | |
Wu RL, Idris AH, Berkowitz NM, Happe M, Gaudinski MR, Buettner C, et al. Low-Dose Subcutaneous or Intravenous Monoclonal Antibody to Prevent Malaria. N Engl J Med. 2022;387(5):397-407. | |
Kayentao K, Ongoiba A, Preston AC, Healy SA, Doumbo S, Doumtabe D, et al. Safety and Efficacy of a Monoclonal Antibody against Malaria in Mali. N Engl J Med. 2022;387(20):1833-42. | |
Larkin HD. Next-Generation Monoclonal Antibody Blocks Malaria in Early Trial. JAMA. 328. United States2022. p. 916-7. | |
Wells T, Donini C. Monoclonal Antibodies for Malaria. N Engl J Med. 2022;387(5):462-5. | |
Menéndez C, D'Alessandro U, ter Kuile FO. Reducing the burden of malaria in pregnancy by preventive strategies. Lancet Infect Dis United States, 2007;7:126–35. |
Online Study Assessment Option
All readers who are qualified doctors or allied medical professionals can now automatically receive 2 Continuing Professional Development credits from FIGO plus a Study Completion Certificate from GLOWM for successfully answering 4 multiple choice questions (randomly selected) based on the study of this chapter.
Medical students can receive the Study Completion Certificate only.
(To find out more about FIGO’s Continuing Professional Development awards programme CLICK HERE)
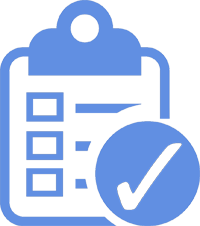