This chapter should be cited as follows:
Santo S, Reis-de-Carvalho C, et al, Glob. libr. women's med.,
ISSN: 1756-2228; DOI 10.3843/GLOWM.412143
The Continuous Textbook of Women’s Medicine Series – Obstetrics Module
Volume 5
Surveillance of fetal well-being
Volume Editor: Professor Diogo Ayres-de-Campos, University of Lisbon, Portugal
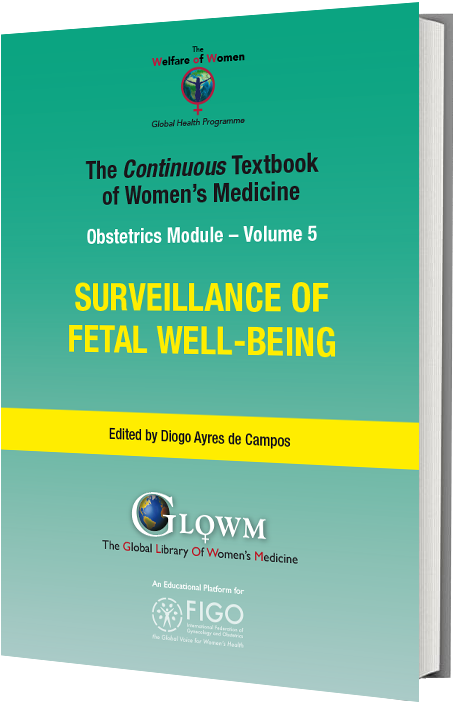
Chapter
Antepartum Cardiotocography
First published: February 2021
Study Assessment Option
By completing 4 multiple-choice questions (randomly selected) after studying this chapter readers can qualify for Continuing Professional Development awards from FIGO plus a Study Completion Certificate from GLOWM
See end of chapter for details
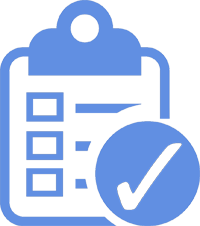
INTRODUCTION
Cardiotocography was commercialized in the late 1960s and rapidly became part of routine obstetric practice.1 Derived from the Greek terms “cardio” (heart) and “tokos” (uterine activity), it continuously acquires and displays fetal heart rate (FHR) and maternal uterine contraction signals.2 The physiological principles behind antepartum cardiotocography are the same as those of intrapartum cardiotocography, the only difference being the occurrence of regular uterine contractions and maternal pushing in the second stage of labor. FHR is an indirect indicator of ongoing fetal oxygenation, evaluating autonomic nervous system control of the heart rate, which is dependent on central nervous system and brainstem function.3 Central nervous system function is represented by the appearance of accelerations coincident with fetal movements, and the occurrence of sleep–activity cycles. FHR also provides information on umbilical cord compression triggering vascular baroreceptors, and sudden hypoxemia triggering vascular chemoreceptors, both of which provoke a parasympathetic response.4 The fine oscillations of the FHR (variability) are modulated by the continuous balance between sympathetic and parasympathetic nervous systems, requiring adequate brainstem function, which is reduced in chronic and acute hypoxia.
In the early days of cardiotocography, the focus of attention was more on the pathophysiological significance of decelerations, and less relevance was given to FHR variability. Consequently, efforts were made to artificially stimulate uterine contractions during antepartum cardiotocography, in order to determine fetal response to these events. Nipple stimulation and exogenous oxytocin were used for this purpose, to cause at least three contractions per 10-minute period, a technique that became known as the “contraction-stress test”.5,6,7 The emergence of multivessel Doppler ultrasound, together with the time-consuming nature of the test, and the impossibility of standardizing the intensity and duration of contractions, led to progressive abandoning of this test. It was also realized that useful information could be extracted solely from the evaluation of FHR accelerations and variability, a concept that became known as the “non-stress test”. Nowadays, it is just known as antepartum cardiotocography.
This chapter reviews the main indications of antepartum cardiotocography and outlines the interpretation and clinical management of an abnormal test.
INDICATIONS
The main aim of antepartum cardiotocography is to evaluate fetal oxygenation in pregnancies complicated by maternal or fetal conditions that can alter placental function. Table 1 displays the main obstetric conditions associated with increased perinatal morbidity and mortality.6,7 Although specific risk factors for adverse perinatal outcomes have been identified, large randomized trials establishing the benefits of antepartum cardiotocography are still lacking.
1
Indications for antepartum cardiotocography.
Diabetes |
Hypertensive disorders of pregnancy |
Poorly controlled hyperthyroidism |
Multiple pregnancy |
Post-term pregnancy |
Systemic lupus erythematosus |
Antiphospholipid syndrome |
Prior fetal demise |
Obesity |
Sickle cell disease |
Alloimmunization |
Fetal growth restriction |
Oligohydramnios |
Decreased fetal activity |
Vaginal bleeding |
Preterm premature rupture of membranes |
Labor induction |
Antepartum cardiotocography is most commonly performed during the third trimester but can usually be acquired from 25 weeks onwards. The frequency and duration of exams depend on the indication and severity of the condition, as well as on gestational age. Intervals range from weekly to three times a day, but in very unstable conditions continuous monitoring may be indicated.
TECHNICAL ASPECTS
In the antepartum period, FHR and uterine contraction signals must be acquired externally, by transducers placed on the maternal abdomen and held in place by elastic bands. FHR is estimated by detecting movements of the heart valves and interventricular septum, using Doppler ultrasound and autocorrelation. The latter is a mathematical algorithm developed in the late 1970s to increase signal quality, which has been incorporated in all modern-day cardiotocography monitors.8 It compares the incoming signal with a sample of pre-recorded ones, measuring overlap in signal areas to establish the FHR. The resulting signal has much less signal loss, but only provides an approximation to true heart rate intervals. External FHR monitoring is also prone to inadvertent monitoring of the maternal heart rate, and to signal artifacts such as double-counting and half-counting. It may also not record fetal cardiac arrhythmias accurately.3
Uterine activity is recorded by a tocodynameter placed over the uterine fundus. This transducer measures increased tensile strength of the uterine wall during contractions. Incorrect placement, reduced tension applied to the elastic band, or increased abdominal adiposity may result in failed or inadequate registration of contractions.8 The technology only provides accurate information on the frequency of contractions. It does not determine their intensity or duration, nor does it evaluate basal uterine tone.
FHR and uterine contraction signals are displayed either on thermal paper or on a computer screen, using scales that may differ between hospitals and countries. The horizontal scale is usually referred to as “paper speed” and can vary between 1 and 3 cm/minute. Paper speeds of 1 cm/min are regularly used throughout the world, but most hospitals in the USA and Japan use 3 cm/min. The vertical FHR scale can also vary between 20 and 30 bpm/cm. It is important that healthcare professionals use paper scales with which they are familiar, as the same tracing may look quite different in different scales, and this may lead to erroneous interpretation.8,9
Evaluation of fetal movements is also frequently seen as part of antepartum cardiotocography. These can be perceived and registered by the mother on an event marker. Alternatively, some cardiotocography monitors incorporate an algorithm extracting low frequency signals from the Doppler spectrum, and thus automatically identify fetal movements.
Cardiotocography acquisition should be performed in the lateral recumbent or half-sitting positions, in a bed or a reclining chair. Maternal supine recumbent positions should be avoided, as they can result in aortocaval compression by the pregnant uterus, thus reducing placental perfusion and fetal oxygenation.
CARDIOTOCOGRAPHY INTERPRETATION
Several guidelines for cardiotocography interpretation can be found in the scientific literature. The International Federation of Obstetrics and Gynecology (FIGO) guidelines of 1987 represent the only international consensus with specific recommendations on antepartum cardiotocography.10 These guidelines were updated in 2015, by the largest international consensus reached in the field, but this time with a specific focus on intrapartum monitoring.11 Given that the pathophysiology of FHR features does not differ between the two periods, the same methodology can be used. The 2015 FIGO guidelines include simple, objective and non-interdependent concepts for cardiotocography interpretation and a three-tier system for tracing classification (Table 2).
Fetal heart rate features
Cardiotocography interpretation begins with the assessment of four basic FHR features: baseline, variability, accelerations and decelerations.8,12,13
Baseline is defined as the mean level of the most horizontal and less oscillatory FHR segments. It is estimated in 10-minute periods and expressed in beats per minute (bpm). In the antepartum period a relatively stable baseline is usually present. In tracings with unstable FHR signals, review of previous segments and evaluation of longer time periods may be necessary to estimate the baseline, in particular to identify the fetal behavioral state of active wakefulness (see below), which can lead to an erroneously high baseline estimation. A fetus has a normal baseline if its value lies between 110 and 160 bpm.8 Preterm fetuses tend to have values towards the upper end of this range and post-term fetuses towards the lower end.12 Tachycardia refers to a baseline value above 160 bpm lasting more than 10 minutes.8 Maternal pyrexia is the most frequent cause, and it may be of extrauterine origin or associated with intrauterine infection. In the initial stages of a non-acute fetal hypoxemia, catecholamine secretion may also result in tachycardia. Other less frequent causes are the administration of beta-agonists, parasympathetic blockers, and fetal arrhythmias such as supraventricular tachycardia and atrial flutter. Bradycardia is a baseline value below 110 bpm lasting more than 10 minutes. Values between 100 and 110 bpm may occur in normal fetuses, especially in post-term pregnancies. Maternal hypothermia, administration of beta-blockers, and fetal arrhythmias such as atrial-ventricular block are other less frequent causes.8
Variability corresponds to the fine oscillations in the FHR signal, and is visually evaluated as the average bandwidth amplitude in 1-minute segments. Normal variability is defined as a bandwidth amplitude of 5–25 bpm. Reduced variability is a bandwidth amplitude below 5 bpm for more than 50 minutes in baseline segments, or for more than 3 minutes during decelerations. It can occur due to brainstem hypoxia and the resulting decreased autonomic system activity, but it can also be due to previous cerebral injury, infection and administration of central nervous system depressants or parasympathetic blockers. During deep sleep, variability is usually in the lower range of normality, but the bandwidth amplitude is seldom under 5 bpm, and this usually lasts less than 50 minutes. There is a high degree of subjectivity in the visual evaluation of variability, and therefore careful re-evaluation or computerized analysis (see below) is recommended in borderline situations.8 Increased variability (saltatory pattern) corresponds to a bandwidth exceeding 25 bpm for more than 30 minutes. The pathophysiology of this pattern is incompletely understood, but it has been described in the initial stages of subacute hypoxia and is presumed to be caused by fetal autonomic instability.
Accelerations are abrupt increases in the FHR above the baseline, of more than 15 bpm amplitude, and lasting more than 15 seconds, but less than 10 minutes. Most accelerations coincide with fetal movements and are a sign of a neurological responsiveness and absent hypoxia. In pregnancies before 32 weeks of gestation the criteria for accelerations is lower (10 bpm amplitude and 10 seconds duration). After 32–34 weeks, with the establishment of fetal behavioral states (see below), accelerations rarely occur during periods of deep sleep, which can last up to 50 minutes.
Decelerations are abrupt decreases in FHR below the baseline with an amplitude of more than 15 bpm and duration exceeding 15 seconds. Decelerations may be classified as early, variable, late or prolonged, according their shape and duration. They are usually associated with uterine contractions, and therefore occur rarely in antepartum cardiotocography.
Early decelerations are shallow, short-lasting, with normal variability within the deceleration, and coincident with contractions. They are believed to be caused by fetal head compression and do not indicate fetal hypoxia.
Variable decelerations (V-shaped) exhibit a rapid drop (onset to nadir in less than 30 seconds), good variability within the deceleration, rapid recovery to the baseline, varying in size, shape and relationship to uterine contractions. They translate a baroreceptor-mediated response to increased arterial pressure, as occurs with umbilical cord compression, and on their own are seldom associated with an important degree of fetal hypoxia.
Late decelerations (U-shaped or with reduced variability) have a gradual onset, gradual return to the baseline (>30 seconds), or reduced variability within the deceleration. When contractions are adequately monitored, late decelerations start more than 20 seconds after the onset of a contraction, the nadir is after the acme, and return to the baseline occurs after the end of the contraction. They are indicative of a chemoreceptor-mediated response to fetal hypoxemia. In the presence of a tracing with no accelerations and reduced variability, the definition of late decelerations also includes those with an amplitude of 10–15 bpm.
Prolonged decelerations last more than 3 minutes and are likely to include a chemoreceptor-mediated component and thus to indicate hypoxemia. Decelerations exceeding 5 minutes, with FHR maintained <80 bpm and reduced variability within the deceleration are usually associated with an episode of acute fetal hypoxia and require emergent intervention.8
The sinusoidal pattern is a regular, smooth, undulating signal, resembling a sine wave, with an amplitude of 5–15 bpm, and a frequency of 3–5 cycles per minute. This pattern lasts more than 30 minutes. The pathophysiological basis for the sinusoidal pattern is incompletely understood, but it usually occurs in association with severe fetal anemia, as is found in anti-D alloimmunization, fetal–maternal hemorrhage, twin-to-twin transfusion syndrome and ruptured vasa previa. Less frequently, it has been described in cases of acute fetal hypoxia, infection, cardiac malformations, hydrocephalus and gastroschisis.11
The pseudo-sinusoidal pattern resembles the sinusoidal pattern but has a more jagged “saw-tooth” appearance, rather than the smooth sine-wave form. The duration seldom exceeds 30 minutes, and is characterized by normal patterns before and after. This pattern has been described after analgesic administration to the mother, and during periods of fetal sucking and other mouth movements. It is sometimes difficult to distinguish the pseudo-sinusoidal from the sinusoidal pattern, and the short duration of the former may be the most important variable to discriminate between the two.
Fetal behavioral states refer to periods of deep sleep (no eye movements), alternating with periods of active sleep (rapid eye movements) and active wakefulness.14 Transitions between these periods become clear after 32–34 weeks, as a consequence of fetal nervous system maturation. The occurrence of different behavioral states is a sign of neurological responsiveness and absent hypoxia. Deep sleep can last up to 50 minutes15 and is associated with a stable baseline, very rare accelerations, and borderline variability. Active sleep is the most frequent behavioral state and is represented by a moderate number of accelerations and normal variability. Active wakefulness is rarer and represented by a large number of accelerations and normal variability. In the latter pattern, accelerations may be so frequent as to cause difficulties in baseline estimation.8
Contractions are bell-shaped gradual increases in uterine activity, followed by roughly symmetric decreases, with 45–120 seconds in total duration.8
Tracing classification
Classification of cardiotocography tracings requires previous evaluation of the FHR features described above. Tracings may be classified into one of three categories: normal, suspicious or pathological,8 according to Table 2.
2
Cardiotocography classification adapted from the 2015 FIGO guidelines.8
Normal | Suspicious | Pathological | |
Baseline | 110–160 bpm | Lacking at least one characteristic of normality, but with no pathological features | <100 bpm |
Variability | 5–25 bpm | Reduced variability Increased variability Sinusoidal pattern | |
Decelerations | No repetitive* decelerations | Repetitive* late or prolonged decelerations for >30 min (or >20 min if reduced variability) Deceleration >5 min | |
Interpretation | No hypoxia/acidosis | Low probability of hypoxia/acidosis | High probability of hypoxia/acidosis |
*Decelerations are repetitive when associated with >50% contractions
A normal tracing has a baseline of 110–160 bpm, variability 5–25 bpm, and no repetitive decelerations. In the antepartum it will also have accelerations. A suspicious tracing lacks one at least one of these characteristics, but has no pathological features. Pathological tracings have one or more of the criteria outlined in Table 2.
Some guidelines classify cardiotocography tracings as reactive or non-reactive. Probably the most commonly used definition for a reactive tracing is the presence of two or more accelerations in a 20-minute period.16 Durations of 30, 40, 50, 60 minutes or even longer have also been proposed.17,18 The required number of accelerations also varies, ranging from two in 10 minutes to two to five in 20 minutes.17
Vibroacoustic stimulation, using an artificial larynx placed on the maternal abdomen, has been used to wake the fetus up from the state of deep sleep, and thus decrease the duration of non-reactive tracings. A systematic review of the scientific literature showed that vibroacoustic stimulation reduces the frequency of non-reactive tracings by about 40% (OR 0.62, 95% CI 0.48–0.81).19 However, the option of maintaining monitoring for longer time periods seems a more physiological alternative, as abnormally prolonged periods of active wakefulness have been reported after vibroacoustic stimulation.
CLINICAL DECISION BASED ON ANTEPARTUM CARDIOTOCOGRAPHY
Several factors need to be considered when interpreting antepartum cardiotocography tracings and determining clinical management: particularly gestational age, medication given to the mother, and the overall clinical picture. Preterm fetuses may show a relatively high baseline, lower variability and lower amplitude of accelerations. Post-term fetuses may show a baseline in the lower limit of normality. In addition to the already mentioned medications affecting FHR features, magnesium sulfate has been reported to reduce variability and accelerations20,21,22,23 and betamethasone to decrease variability, with a peak effect during the second day.24,25,26,27 Integrating cardiotocography interpretation into the overall clinical picture is essential for adequate management. Maternal condition, gestational age, and ultrasound results are other crucial aspects of the decision process.
Antepartum cardiotocography is reported to have a 99.9% negative predictive value,7 meaning that a normal tracing almost completely excludes ongoing fetal hypoxia. In the past, expectations were raised that a normal antepartum cardiotocography could predict fetal outcome for several days or even weeks following the test. It is now well known that these expectations were unrealistic, given the unpredictable course of intrauterine oxygenation. Naturally, adverse outcomes related to acute or subacute events, such as placental abruption, umbilical cord prolapse, uterine rupture, etc. cannot be predicted. Likewise, prediction of events following the onset of labor is unrealistic.
In cases of progressive placental insufficiency, the fetal brain-sparing effect protects the central nervous system and brainstem from the effects of chronic hypoxemia until late in its course, so cardiotocography changes are therefore late markers of disease progress. These situations need to be monitored together with multivessel fetal Doppler (see corresponding chapter) providing an earlier detection of placental function abnormalities. Cardiotocography is mainly useful to determine the decision to deliver, with the strongest evidence available for computerized cardiotocography analysis (see below).
Interpretation of antepartum cardiotocography relies heavily on evaluation of variability, a feature that is difficult to assess with the naked eye. High false positive rates have been reported in the prediction of several adverse outcomes, including metabolic acidosis, low Apgar scores, and neonatal intensive care unit admissions. This evaluation also suffered from the lack of agreement on the definition of adverse outcomes existing in the late part of the last century, i.e. baseline, variability, accelerations, deceleration, etc.
A meta-analysis of six randomized controlled trials comparing the use of antepartum cardiotocography with no use, showed no differences in perinatal mortality (RR 2.05, 95% CI 0.95–4.42) or potentially preventable deaths (RR 2.46, 95% CI 0.96–6.30). Despite these results, antepartum cardiotocography continues to be extensively used in high-resource countries, translating how this evidence is considered in routine clinical practice. This is reflected in the guidelines of several international scientific societies that recommend the use of antepartum cardiotocography in high-risk pregnancies.
Because it does not require a lot of expertise for acquisition, it is non-invasive, patient friendly, and lacks contraindications, antepartum cardiotocography has become part of antepartum care in many countries. However, expertise is required for tracing interpretation, as well as for integrating results in the overall clinical picture, and for deciding management. A normal cardiotocography is usually reassuring but an abnormal one may cause anxiety, unnecessary intervention, and an abnormal outcome. Guaranteeing access to expert analysis is therefore required for adequate use of the technology.7
COMPUTERIZED ANALYSIS OF ANTEPARTUM CARDIOTOCOGRAPHY
Cardiotocography interpretation is known to suffer from a high intra-observer and interobserver disagreement, both in evaluation of individual cardiotocography features and in overall classification.28,29,30 Agreement is particularly low for evaluation of variability, decelerations and classification of tracings as suspicious or pathological. Use of different guidelines for cardiotocography interpretation has also been shown to play a role in the magnitude of disagreement.31
Computerized analysis of cardiotocography was developed in the 1980s and 1990s, as an effort to overcome the limited reproducibility of visual analysis. Complex mathematical algorithms estimate the FHR baseline, identify accelerations and decelerations and quantity variability. The latter aspect is particularly important, as more objective information can be obtained from a detailed analysis of long-term and beat-to-beat intervals (short-term variability).
The Dawes/Redman system was developed in Oxford in the late 1970s and commercialized in 1989,32 with a major upgrade being conducted in 1994.33 Signal reduction to 3.75 second epochs is performed, “short-term variation” is calculated as the average difference between successive epochs, and “long-term variation” as the difference between the highest and lowest epoch values in 1-minute segments, excluding decelerations. The system has been compared with experts21,34,35 and its predictive capacity was extensively studied.32,33,35,36,37,38,39 Long-term variation correlated well with hypoxemia documented by cordocentesis,40 but limited sensitivity was obtained in detection of moderate to severe hypoxemia.41 Reduced short-term variation achieved a high accuracy in prediction of newborn acidemia in fetuses with growth-restriction submitted to cesarean section shortly after the exam,42 but these results have not been replicated.43
Four randomized controlled trials compared the Dawes/Redman system with visual analysis and found as the only significant differences: better signal quality,44 a reduction in the duration of registrations,45,46 and a reduction in the number of additional exams.46 Meta-analysis of two of these studies showed a significant reduction in perinatal mortality with the Dawes/Redman system (RR 0.20, 95% CI 0.04–0.88, 0.9% versus 4.2%, 469 women). However, there was no significant difference in potentially preventable deaths (RR 0.23, 95% CI 0.04–1.29, 469 women), cesarean deliveries (RR 0.87, 95% CI 0.61–1.24, 63% versus 72%, 59 women), or low 5-minute Apgars (RR 1.31, 95% CI 0.30–5.74, 469 women).47 Delivery based on reduced short-term variation was compared with ductus venosus changes in a multicenter randomized controlled trial evaluating growth-restricted fetuses between 26 and 32 weeks, and similar proportions of survival without neurodevelopmental impairment were found in both groups.
This SisPorto system was developed at the University of Porto, Portugal. Cardiotocography analysis follows the 2015 FIGO guidelines, and no signal reduction is performed.48 The system uses complex mathematical algorithms to identify basic cardiotocography features and provide real-time visual and sound alerts for cardiotocography abnormalities. The system’s analysis was compared with a consensus of experts, with very favorable results. A high accuracy was found in the capacity to predict low Apgar scores and acidemia, when analysis was performed shortly before cesarean delivery. Use of the system led to an increase in signal quality and decrease in signal loss in a tertiary care hospital. Normality references for short- and long-term variability were established throughout gestation. Small-for-gestational-age fetuses showed lower short- and long-term variability, and lower number of accelerations.49
CONCLUSIONS
The main goal of antepartum cardiotocography is to identify fetuses with ongoing hypoxemia that will benefit from early intervention. It is recommended for fetal surveillance in high-risk pregnancies, although cardiotocography changes appear late in the course of progressive placental insufficiency and there is limited evidence on its effectiveness. Antepartum cardiotocography has a negative predictive value, i.e. a normal tracing almost excludes the occurrence of ongoing fetal hypoxia. Interpretation of tracings with abnormal characteristics requires expertise, as does subsequent clinical decision making, which should take into account other clinical aspects. Computerized analysis of cardiotocography provides a more objective quantification of variability and its use in growth-restricted fetuses has been shown to result in decreased perinatal mortality.
PRACTICE RECOMMENDATIONS
Cardiotocography continuously acquires and displays fetal heart rate (FHR) and maternal uterine contractions and is an indirect indicator of ongoing fetal oxygenation.
- The physiological principles behind antepartum cardiotocography are the same as those of intrapartum cardiotocography and 2015 FIGO guidelines may be used for antenatal cardiotocography interpretation.
- Antepartum cardiotocography has been recommended in high-risk pregnancies. It is commonly performed during the third trimester. The frequency and duration of exams depends on gestational age and on the indication and severity of the condition.
- Antepartum cardiotocography has a high negative predictive value for fetal hypoxia.
- Integrating cardiotocography interpretation in the overall clinical situation is essential for adequate management decisions, as it is just an additional test.
CONFLICTS OF INTEREST
Author(s) statement awaited.
Feedback
Publishers’ note: We are constantly trying to update and enhance chapters in this Series. So if you have any constructive comments about this chapter please provide them to us by selecting the "Your Feedback" link in the left-hand column.
REFERENCES
Nunes I, Ayres-de-Campos D, Figueiredo C, Bernardes J. An overview of central fetal monitoring systems in labour. J Perinat Med 2013;41:93–9. | |
Queensland Health. Intrapartum fetal surveillance (IFS). Queens Matern Neonatal Clin Guidel 2015. | |
Pinas A, Chandraharan E. Continuous cardiotocography during labour: Analysis, classification and management. Best Pract Res Clin Obstet Gynaecol 2016;30:33–47. | |
Ayres-de-Campos D, Arulkumaran S. FIGO consensus guidelines on intrapartum fetal monitoring : Introduction. Int J Gynecol Obstet 2015;131:3–4. | |
Ray M, Freeman R, Pine S, Hesselgesser R. Clinical experience with the oxytocin challenge test. Am J Obstet Gynecol 1972;114:1–9. | |
Liston R, Sawchuck D, Young D, Brassard N, Campbell K, Davies G, et al. Fetal Health Surveillance: Antepartum and Intrapartum Consensus Guideline. J Obstet Gynaecol Canada 2007;29:S3–4. | |
ACOG. Antepartum Fetal Surveillance. Obstet Gynecol 2014;123:1118–32. | |
Ayres-de-Campos D, Spong CY, Chandraharan E. FIGO consensus guidelines on intrapartum fetal monitoring: Cardiotocography. Int J Gynecol Obstet 2015;131:13–24. | |
Brocklehurst P. A study of an intelligent system to support decision making in the management of labour using the cardiotocograph – the INFANT study protocol. BMC Pregnancy Childbirth 2016;16:10. | |
FIGO subcommittee on Standards on Perinatal Medicine. Guidelines for the use of fetal monitoring. Int J Gynaecol Obstet 1987;25:159–67 | |
Rajasingam D, Harding K. NICE’s draft guideline on intrapartum care. BMJ 2014;348:g4279. | |
National Institute for Health and Care Excellence (NICE). Intrapartum care: NICE guideline CG190 Interpretation of cardiotocograph traces. 2017;190. | |
Nijhuis JG, Prechtl HFR, Martin CB, Bots RSGM. Are there behavioural states in the human fetus? Early Hum Dev 1982;6:177–95. | |
Suwanrath C, Suntharasaj T. Sleep-wake cycles in normal fetuses. Arch Gynecol Obstet 2010;281:449–54. | |
Evertson LR, Gauthier RJ, Schifrin BS, Paul RH. Antepartum fetal heart rate testing. I. Evolution of the nonstress test. Am J Obstet Gynecol 1979;133:29–33. | |
Devoe LD, Castillo RA, Sherline DM. The nonstress test as a diagnostic test: A critical reappraisal. Am J Obstet Gynecol 1985;152:1047–53. | |
Brown R, Patrick J. The nonstress test: How long is enough? Am J Obstet Gynecol 1981;141:646–51. | |
Kh T, Smyth R. Fetal vibroacoustic stimulation for facilitation of tests of fetal wellbeing. Cochrane Database Syst Rev 2013:CD002963. | |
Guzman ER, Conley M, Stewart R, Ivan J, Pitter M, Kappy K. Phenytoin and magnesium sulfate effects on fetal heart rate tracings assessed by computer analysis. Obstet Gynecol 1993;82:375–9. | |
Hiett AK, Devoe LD, Brown HL, Watson J. Effect of Magnesium on Fetal Heart Rate Variability Using Computer Analysis. Am J Perinatol 1995;12:259–61. | |
Atkinson MW, Belfort MA, Saade GR, Moise KJ. The relation between magnesium sulfate therapy and fetal heart rate variability. Obstet Gynecol 1994;83:967–70. | |
Hallak M, Martinez-Poyer J, Kruger ML, Hassan S, Blackwell SC, Sorokin Y. The effect of magnesium sulfate on fetal heart rate parameters: A randomized, placebo-controlled trial. American Journal of Obstetrics and Gynecology 1999;181:1122–7. | |
Derks JB, Mulder EJH, Visser GHA. The effects of maternal betamethasone administration on the fetus. BJOG 1995;102:40–6. | |
Mulder EJH, de Heus R, Visser GH. Antenatal corticosteroid therapy: short-term effects on fetal behaviour and haemodynamics. Semin Fetal Neonatal Med 2009;14:151–6. | |
Senat M V., Minoui S, Multon O, Fernandez H, Frydman R, Ville Y. Effect of dexamethasone and betamethasone on fetal heart rate variability in preterm labour: a randomised study. Br J Obstet Gynaecol 1998;105:749–55. | |
Rotmensch S, Celentano C, Liberati M, Sadan O, Glezerman M. The effect of antenatal steroid administration on the fetal response to vibroacoustic stimulation. Acta Obstet Gynecol Scand 1999;78:847–51. | |
Ayres-de-Campos D, Bernardes J, Marsal K, Nickelsen C, Makarainen L, Banfield P, et al. Can the reproducibility of fetal heart rate baseline estimation be improved? Eur J Obstet Gynecol Reprod Biol 2004;112:49–54. | |
Ayres-de-Campos D, Bernardes J. Early, variable and late decelerations: can a consensus be reached in their identification? Int J Gynaecol Obstet 1999;65:305–6. | |
Ayres-de-Campos D, Bernardes J, Costa-Pereira A, Pereira-Leite L. Inconsistencies in classification by experts of cardiotocograms and subsequent clinical decision. Br J Obstet Gynaecol 1999;106:1307–10. | |
Santo S, Ayres-de-Campos D, Costa-Santos C, Schnettler W, Ugwumadu A, Da Graça LM, et al. Agreement and accuracy using the FIGO, ACOG and NICE cardiotocography interpretation guidelines. Acta Obstet Gynecol Scand 2017;96:166–75. | |
Henson G, Dawes GS, Redman CWG. Characterization of the reduced heart rate variation in growth‐retarded fetuses. BJOG 1984;91:751–5. | |
Dawes GS, Moulden M, Redman CWG. Improvements in computerized fetal heart rate analysis antepartum. J Perinat Med 1996;24:25–36. | |
Bracero LA, Roshanfekr D, Byrne DW. Analysis of antepartum fetal heart rate tracing by physician and computer. J Matern Fetal Med 2000;9:181–5. | |
Seliger G, Stenzel A, Kowalski EM, Hoyer D, Nowack S, Seeger S, et al. Evaluation of standardized, computerized Dawes/ Redman heart-rate analysis based on different recording methods and in relation to fetal beat-to-beat heart rate variability. J Perinat Med 2015;1:785–792. | |
Schneider KTM, Beckmann MW, German Society of Gynecology, German Society of Prenatal Medicine, German Society of Perinatal Medicine (DGPM). S1-guideline on the use of CTG during pregnancy and labor. Geburtshilfe Frauenheilkd 2014;74:721–32. | |
Guzman ER, Vintzileos AM, Martins M, Benito C, Houlihan C, Hanley M. The efficacy of individual computer heart rate indices in detecting acidemia at birth in growth-restricted fetuses. Obstet Gynecol 1996;87:969–74. | |
Cheng LC, Gibb DMF, Ajayi RA, Soothill PW. A comparison between computerised (mean range) and clinical visual cardiotocographic assessment. BJOG 1992;99:817–20. | |
Rei M, Tavares S, Pinto P, Machado A, Monteiro S, Costa-Santos C, et al. Interobserver agreement in CTG interpretation using the 2015 FIGO guidelines for intrapartum fetal monitoring. Eur J Obstet Gynecol 2016;205:27–31. | |
Visser GHA, Sadovsky G, Nicolaides KH. Antepartum heart rate patterns in small-for-gestational-age third-trimester fetuses: Correlations with blood gas values obtained at cordocentesis. Am J Obstet Gynecol 1990;162:698–703. | |
Nicolaides KH, Economides DL, Soothill PW. Blood gases, pH, and lactate in appropriate- and small-for-gestational-age fetuses. Am J Obstet Gynecol 1989;161:996–1001. | |
Guzman E, Martins M, Hanley M, Vintzileos A, Benito C, Houlihan C. The Efficacy of Individual Computer Heart Rate Indices in Detecting Acidemia at Birth in Growth-Restricted Fetuses. Obstet Gynecol 2002;87:969–74. | |
Anceschi MM, Piazze JJ, Vozzi G, Ruozi Berretta A, Figliolini C, Vigna R, et al. Antepartum computerized CTG and neonatal acid-base status at birth. Int J Gynecol Obstet 1999;65:267–72. | |
Dawes GS, Lobb M, Moulden M, Redman CWG, Wheeler T. Antenatal cardiotocogram quality and interpretation using computers. BJOG 2014;121:2–8. | |
Van Geijn HP. Antepartum assessment of fetal condition by means of cardiotocography. In: Current Obstetrics and Gynaecology 1997. | |
Bracero LA, Morgan S, Byrne DW. Comparison of visual and computerized interpretation of nonstress test results in a randomized controlled trial. Am J Obstet Gynecol 1999;181:1254–8. | |
Grivell RM, Alfirevic Z, Gyte GML DD. Antenatal cardiotocography for fetal assessment. Cochrane database Syst Rev 2015;(9. Art. No.: CD007863). | |
Ayres-de-Campos D, Rei M, Nunes I, Sousa P, Bernardes J. SisPorto 4.0 – computer analysis following the 2015 FIGO Guidelines for intrapartum fetal monitoring. J Matern Neonatal Med 2016;3:1–15. | |
Costa MA, Ayres-de-Campos D, Machado AP, Santos CC, Bernardes J. Comparison of a computer system evaluation of intrapartum cardiotocographic events and a consensus of clinicians. J Perinat Med 2010;38:191–5. |
Online Study Assessment Option
All readers who are qualified doctors or allied medical professionals can now automatically receive 2 Continuing Professional Development credits from FIGO plus a Study Completion Certificate from GLOWM for successfully answering 4 multiple choice questions (randomly selected) based on the study of this chapter.
Medical students can receive the Study Completion Certificate only.
(To find out more about FIGO’s Continuing Professional Development awards programme CLICK HERE)
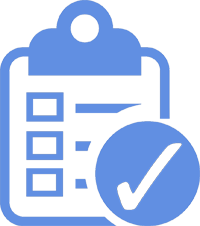