This chapter should be cited as follows:
Morton A, Glob. libr. women's med.,
ISSN: 1756-2228; DOI 10.3843/GLOWM.413143
The Continuous Textbook of Women’s Medicine Series – Obstetrics Module
Volume 8
Maternal medical health and disorders in pregnancy
Volume Editor:
Dr Kenneth K Chen, Alpert Medical School of Brown University, USA
Originating Editor: Professor Sandra Lowe
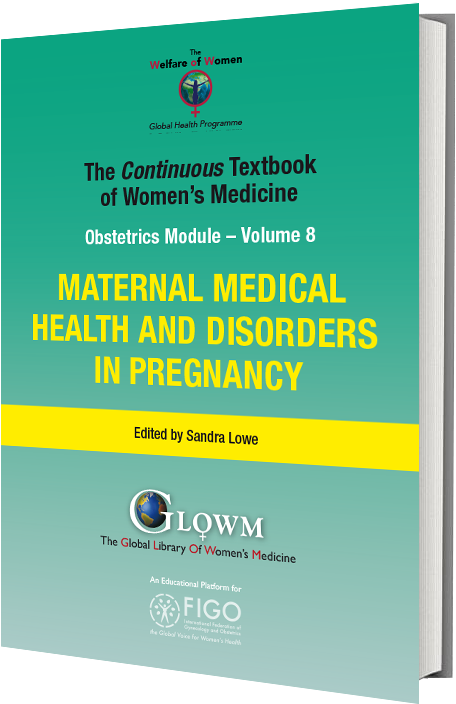
Chapter
Nutritional Anemia
First published: February 2021
Study Assessment Option
By completing 4 multiple-choice questions (randomly selected) after studying this chapter readers can qualify for Continuing Professional Development awards from FIGO plus a Study Completion Certificate from GLOWM
See end of chapter for details
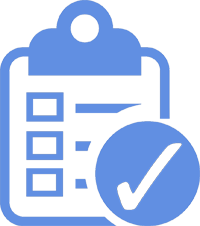
INTRODUCTION
Anemia affects an estimated 30% of women of child-bearing age, and more than 40% of pregnant women worldwide. The highest prevalence of anemia in pregnant women is in South-East Asia (48.7%) and Africa (46.3%).1
Anemia in pregnancy is defined by the World Health Organization (WHO) as hemoglobin (Hb) less than 110 g/dL in the first and third trimesters, and less than 105 g/dL in the second trimester.
Micronutrient deficiencies are common in women of reproductive age, particularly in developing countries. A systematic review of data from 2005 to 2015 of reproductive age women in Ethiopia, Kenya, Nigeria and South Africa found the prevalence of anemia, iron deficiency, iron deficiency anemia, folate deficiency, zinc, vitamin A and iodine deficiencies to be 18–51%, 9–18%, 10%, 46%, 34%, 4–22% and 22–55%, respectively.2 The corresponding prevalence rates in pregnant women were 32–62%, 19–61%, 9–47%, 3–12%, 46–76%, 21–48% and 87%. Women of reproductive age in developing countries are particularly susceptible to micronutrient deficiencies due to women prioritizing the health of other family members and, thus, inequitable food distribution within households, lack of availability of food, lack of dietary diversity, and endemic infectious diseases. As a result, women may enter pregnancy with micronutrient deficiencies, which may be further exacerbated by the demands of pregnancy.
IRON DEFICIENCY
Iron deficiency is the most common cause of anemia in pregnancy, accounting for up to 75% of cases. In addition, iron deficiency anemia (IDA) affects 10% of postpartum women. It is estimated that an additional 500 mg of iron is required during pregnancy for expansion of red cell mass, 300–350 mg for the growing fetus and placenta, as well as 250 mg for peripartum blood loss. Physiologic requirements for iron absorbed are estimated to be 0.8 mg/day in first trimester, 5 mg/day in second trimester and 6 mg/day in third trimester.3 A typical Western diet provides only 50% of daily iron requirements for pregnant women.4 A significant proportion of women have inadequate iron stores in early pregnancy even in the absence of anemia. An observational study of 102 non-anemic first trimester women in the USA found that 37% were iron deficient based upon a transferrin saturation of less than 20%.5 Fourteen per cent had ferritin levels <30 ng/mL, 6% had ferritin levels <25 ng/mL, and 5% had ferritin levels <20 ng/mL. A recent study in Indian women found the prevalence of iron deficiency was 91% in first trimester.6
Risk factors for iron deficiency in pregnancy include low dietary intake, malabsorption, blood loss, adolescent pregnancy, obesity, gestational diabetes, hypertension, higher parity, a short interpartum period, and carrying multiple fetuses.
DIAGNOSING IRON DEFICIENCY
Serum ferritin is usually adequate for the diagnosis of iron deficiency, with the exception of where there is active inflammation, as ferritin acts as an acute phase reactant. Diagnostic thresholds for iron deficiency in pregnancy vary significantly. While WHO defines iron deficiency anemia based on a serum ferritin <15 ng/mL, pregnant women with serum ferritin levels <30 ng/mL are considered to be at risk for IDA in the United Kingdom, and in Denmark it is suggested that women with serum ferritin <70 g/mL be treated with iron supplements.7
Studies correlating the presence or absence of stainable marrow iron with serum ferritin indicated that a level of <30 ng/mL has a 92% sensitivity and 98% specificity for diagnosing iron deficiency, whereas a serum ferritin <10 ng/ml has only a 25% sensitivity in detecting iron deficiency.8,9
In the setting of acute inflammation measurement of transferrin saturation may be useful. Transferrin saturation <16% is considered a marker of functional iron deficiency.
The use of microcytosis in screening will underestimate the prevalence of IDA, as a fall in Hb commonly antedates the fall in mean corpuscular volume.10 Microcytosis was present in only 27.5–65% of patients with iron deficiency.11,12 Soluble transferrin receptor levels increase in iron deficiency, and are unaffected by inflammation. A meta-analysis of ten studies found that soluble transferrin receptor levels had a sensitivity of 86% and a specificity of 75% in detecting iron deficiency.13 The assay is not standardized and not widely available. Hepcidin regulates systemic iron bioavailability, determining how well oral iron is absorbed. Levels of hepcidin fall during pregnancy, women with undetectable levels transferring more maternally ingested iron to their fetus than women with detectable hepcidin. The utility of hepcidin levels as a biomarker of iron deficiency is being evaluated, one recent study suggesting that serum hepcidin is superior to Hb, serum iron, serum ferritin, transferrin saturation, and transferrin iron binding capacity as an indicator of IDA in pregnant women.14 Hepcidin may be particularly useful in detecting iron deficiency in the setting of infectious or inflammatory disease.
Based upon measures of erythrocyte and reticulocyte indices including reticulocyte Hb content and percentages of hypochromic erythrocytes and microcytic erythrocytes, Demmers et al. concluded that in contrast to iron-deficient nonpregnant women, low ferritin concentrations in pregnant women are not associated with functional iron deficiency.7 Iron metabolism might be differentially regulated for optimal fetal growth and development despite low maternal iron stores.
Portable point-of-care devices (POCD) for the assessment of Hb levels from capillary blood may be useful in remote areas.15,16 Detection of iron deficiency using POCD may also be useful particularly in remote areas and developing countries. A mobile phone device-coupled tool measuring serum ferritin using fingerprick blood samples displayed a correlation of 0.92 with a sensitivity of greater than 90% for predicting iron deficiency (ferritin <15 ng/mL) compared with a laboratory standard IMMULITE analysis of whole blood samples.17 Similarly an immunochromatographic assay-based point-of-care screening device quantified soluble transferrin receptor from a drop of serum within a few minutes with a correlation of 0.93 compared with ELISA of archived serum samples demonstrating its potential for point-of-care assessment of iron status.18 Additionally erythrocyte zinc-protoporphyrin/heme ratio was found to be a valid point-of-care biomarker to diagnose iron deficiency anemia.19
PREVENTION OF IRON DEFICIENCY
Appropriate dietary advice should be given to all women regarding healthy eating. Appropriate surveillance and treatment should be given regarding endemic infections that may cause anemia (e.g. hookworm, malaria).
Fortification of food with iron has met with a number of barriers. Fortified foods are often financially unavailable to the poorest segments of the population who have the greatest risk of micronutrient deficiencies.20 Food choices for fortification need to be chosen carefully in order to deliver greatest benefit to those individuals at highest risk. Coverage surveys in four African countries found fortification of wheat, maize and semolina flours contributed only 0–13% of recommended iron intake in women of reproductive age.21 A study in the Solomon Islands found that the potential benefit of fortification of wheat flour was likely to be limited to urban populations, and that fortification of rice would contribute significantly to the intake of iron, folic acid and zinc by women of reproductive age.22 Universal fortification of food with iron may place individuals with hemochromatosis at greater risk. Denmark and Sweden, however, ceased iron fortification of foods in 1987 and 1994, respectively, because of both a lack of evidence of benefit and concerns regarding hemochromatosis. The combined allele frequency of the H63D and C282Y mutations for hemochromatosis is only 2.6% in Africa, 1.9% in South-East Asia and 8.6% in the Indian subcontinent, compared with an allele frequency of up to 30% in Northern European populations, suggesting the benefit far outweighs risks in those areas where iron deficiency is highly prevalent.23
Iodized salt is an ideal carrier for food fortification as it is universally consumed independent of economic status.24 Previous attempts to fortify food with both iodine and iron together have been limited by iron negating the effect of iodine. Microencapsulation based technology enables the addition of iron without degradation or interaction, and currently double-fortified salt is reaching 60 million people in three states in India.
A stable quadruple fortification of salt with iodine, iron, folic acid and vitamin B12 that could provide up to 50% of the recommended dietary allowance (RDA) of folic acid and iron, and 100% of RDA of vitamin B12 and iodine has been pilot tested with high consumer acceptability.25 It is estimated this formulation would cost less than US$ 0.20 per person per annum.
Fortification of water with iron and vitamin C has been effective in improving ferritin and Hb in a number of studies.26,27,28,29
Because of the importance of adequate pre-pregnancy iron stores, WHO guidelines recommend once-weekly supplementation with 60 mg elemental iron and 2.8 mg folic acid in all non-pregnant women of reproductive age living in regions with a greater than 20% prevalence of anemia (most developing countries). This together with regular deworming has been shown to be effective in increasing iron stores, stabilizing Hb and reducing soil transmitted helminth infections over periods of several years.30 The equivalent of 60 mg of elemental iron is 300 mg of ferrous sulfate heptahydrate, 180 mg ferrous fumarate or 500 mg ferrous gluconate.
In countries where anemia prevalence is over 40%, WHO recommends daily elemental iron supplementation of 60 mg in pregnant women together with folic acid 0.4 mg.31 Routine supplementation with 15–30 mg/day of elemental iron is recommended in pregnancy in North America.4 Weekly therapy with 120 mg of iron appears to be an effective and safe alternative to daily therapy.32 UK guidelines differ in that routine supplementation of all pregnant women is not recommended, instead screening for anemia is performed in early pregnancy and at 26 weeks' gestation.33 The bioavailability of non-heme iron in foods of plant origin is low, and vegetarian women should be offered iron supplements at a recommended dosage of 30 mg/day.
In a study in which 133 pregnant African women were injected with 5-ml iron dextran complex in each buttock at their first antenatal visit, 84% of the treated group showed an improvement in Hb level (mean rise 1.025 g/dL) compared with 56% of women in a control group (mean rise 0.26 g/dL).34
Oral iron therapy commonly causes constipation, diarrhea and nausea, and non-adherence rates of prescribed oral supplements in pregnancy have been reported to be 27–46%.35 Recommendations for universal iron supplementation have not resulted in a consistent reduction in the incidence of iron deficiency anemia in pregnancy. Intermittent supplementation (2–3 times a week on non-consecutive days) produced similar maternal and infant outcomes to daily supplementation.
Iron salt preparations containing equivalent amounts of elemental iron have similar rates of side-effects.36 Controlled-release iron formulations have lower rates of epigastric pain and nausea, but no difference in discontinuation rates compared with ferrous sulfate.37,38 Absorption of iron may be increased by concurrent administration of vitamin C. Oral iron is likely to be ineffective in the setting of inflammatory bowel disease, and not absorbed in women who have undergone bypass bariatric surgery.
It is unclear as to whether iron supplementation in malaria endemic areas may increase the risk of infection.39 A 2015 systematic review and meta-analysis of pregnancy concluded that iron supplementation in pregnancy was associated with a temporal increase in Plasmodium vivax infections without a clear effect on Plasmodium falciparum risk.40 A 2018 review of clinical trials, however, concluded that iron supplementation in pregnancy did not increase the risk of malaria.41 In malaria-endemic areas, iron supplementation should occur in conjunction with appropriate malaria prevention, diagnosis, and treatment measures.
TREATMENT OF IRON DEFICIENCY
Where a woman is diagnosed with anemia in pregnancy, the daily dose of oral elemental iron should be increased to 120 mg per day until Hb is at least 110 g/dL.
A study comparing intramuscular administration of iron dextran (250 mg thrice weekly) to pregnant women with IDA resulted in more rapid improvement in Hb at 2, 4 and 6 weeks than oral ferrous sulfate (200 mg 3 times per day with vitamin C).42 The relative cost of parenteral iron dextran treatment was 4–8 times that of oral ferrous sulfate. In addition, intramuscular therapy required transport of the pregnant woman to and from the local hospital for administration in case of anaphylaxis, and was associated with pain at the injection site in 17%, skin staining in 3%, nausea in 10%, and headache in 7%. Intramuscular iron is therefore not recommended as first-line therapy.
A meta-analysis revealed pregnant women with anemia are 2.7 times more likely to achieve their target Hb with iron infusion (FeI) than oral iron, and to do so more rapidly and with less risk of adverse effects.43 Systematic reviews of oral and intravenous iron therapy for iron deficiency anemia in pregnancy demonstrated significant improvement in hematologic parameters though no improvement in clinically relevant outcomes.44,45 A possible contributor to the failure of FeI to improve maternal and fetal outcomes is the late administration – recent retrospective observational Australian studies reported administration of FeI to healthy women at a mean gestation of 34.6 weeks.46,47 FeI is usually avoided in first trimester because of uncertainty regarding teratogenicity.48 Twenty-two cases of intravenous iron administration in healthy women in first trimester have been described without adverse pregnancy outcome.49,50 Additionally, more than 600 pregnancies have been reported in women receiving chronic hemodialysis. Individuals being managed with hemodialysis typically receive 100 mg of intravenous iron weekly. The mean time at which pregnancy is diagnosed in women receiving chronic hemodialysis is 8 weeks, with the fetal malformation rate the same as in the general population, suggesting that intravenous iron in doses used for dialysis patients is not teratogenic in human pregnancy.
FeI products consist of a polynuclear iron core with a surrounding shell of carbohydrate for stability. In contrast to the high rate of adverse reactions to high molecular weight iron dextrans, current formulations have a low incidence of side-effects. The risk of major infusion reactions due to activation of the complement system has been estimated to be 1 in 200,000.51 Minor reactions requiring slowing of the infusion rate occur in 1 in 200. No increased risk of infection or precipitation of cardiac failure has been reported. Ferric carboxymaltose may be associated with hypophosphatemia in up to 50% of patients regularly tested post FeI.52 Onset occurred approximately 1 week after FeI, peaking in frequency at 2 weeks post FeI, and persisted for up to 5 weeks. The vast majority of cases of hypophosphatemia are asymptomatic – only nine cases of severe hypophosphatemia with musculoskeletal complications having been described to date, none of these in pregnancy. FeI products appear to have equivalent efficacy and safety. Formulations that may be given as a single infusion, such as ferric carboxymaltose, iron polymaltose and low molecular weight iron dextran, may be preferable.
Response to iron therapy is usually seen as a reticulocytosis within 1 week, a rise in Hb within 2 weeks, and an increase in serum ferritin within 3 weeks.
Lactoferrin is an iron-binding protein present in large quantities in colostrum, breast milk, external secretions and leucocytes. Its functions include gastrointestinal absorption of iron as well as antimicrobial, antioxidant, anti-inflammatory and anti-neoplastic activity. Lactoferrin has been approved by the FDA and European Food Safety Authority as a supplement in food products. Lactoferrin is taken orally twice a day. A meta-analysis of randomized trials found lactoferrin was significantly more effective than oral ferrous salts in improving Hb level after 4 weeks in pregnant women with moderate iron deficiency anemia, and was better tolerated.53 In a randomized controlled trial lactoferrin with health education resulted in a similar improvement in anemia to total dose infusion of iron dextran 4 weeks after treatment.54 The cost of lactoferrin may be a significant barrier to its widespread use. In an Egyptian study the cost of 4 weeks of lactoferrin therapy was $7.20US compared with $0.41US for treatment with ferrous sulfate.55
Several animal studies have demonstrated significant improvement in serum iron and Hb levels in IDA with the use of dissolving microneedle patches containing ferric pyrophosphate and iron dextran.56,57,58,59 Transdermal delivery of iron may be an alternative to intravenous and oral therapy in remote areas. Nasal delivery of nano-liposome encapsulated ferric ammonium citrate has been used to increase iron content in the brain of the rat without affecting the respiratory system.60
FOLATE DEFICIENCY
Folate deficiency complicates approximately 30% of pregnancies worldwide.61 The folic acid requirement during pregnancy increases by 50% to 150 mg per day. Dietary folate is absorbed in the jejunum. The total body content of folate is estimated to be 15–30 mg, half of these being in the liver, the remainder in blood and body tissues.62 Folate stores last approximately 3 months before deficiency occurs.
Folic acid fortification of foods (providing approximately 163 mg/day in the US) has resulted in a reduction in neural tube defects (NTD) in many countries. The neural tube closes around day 26 of gestation. Causes of folic acid deficiency include diets high in organic foods or low in carbohydrate, interference of absorption due to medications or medical conditions (celiac disease, inflammatory bowel disease, gastric bypass surgery), liver and renal disease, and alcohol excess.
Most pregnant women with folate deficiency anemia do not manifest macrocytosis.63 Red cell folate levels are a more accurate measure of folic acid deficiency than serum folate. A POCD using a fluorescence lateral flow assay to measure serum folate has been described and may be useful for measuring levels in areas remote to laboratory facilities.64
For the prevention of neural tube defects empiric supplementation with 0.4 mg folic acid per day is recommended from 3 months prior to attempting conception continuing throughout pregnancy and breastfeeding. In women with medical conditions predisposing to low folate levels as above, pregestational diabetes, medications that may lower folic acid levels (e.g. sulfasalazine, trimethoprim, triamterene), or a family history of NTD in second or third degree relatives, a dose of 1 mg folic acid per day is recommended pre-conception/first trimester, reducing to 0.4 mg per day at 12 weeks' gestation and continuing until cessation of breastfeeding. With maternal therapy with carbamazepine or sodium valproate, a parental or previous offspring history of NTD, or sickle cell anemia or thalassemia, 4–5 mg folic acid per day is recommended preconception/first trimester. A preconception/first trimester 1 mg/day dose is also recommended where there is a family history of cleft lip/palate, congenital heart defects, limb defects, urinary tract abnormalities and congenital hydrocephalus, as these may also represent folate-sensitive congenital malformations.
In the treatment of anemia due to folate deficiency, replacement may be achieved with a daily 5 mg oral dose for 4 months or 0.5–1 mg two or three times daily.65 Holotranscobalamin levels should always be measured prior to treating megaloblastic anemia, as therapy with folic acid may mask diagnosis of vitamin B12 deficiency increasing the risk of neurologic or hematologic complications.
COBALAMIN DEFICIENCY
Cobalamin deficiency is far less common than folate deficiency in pregnancy as substantial amounts of vitamin B12 are stored in the liver, enough to maintain supply for 3–6 years. Primary dietary sources of vitamin B12 are meats, fish, shellfish, and dairy products, absorption of B12 occurring in the terminal ileum. Dietary deficiency of B12 is common in vegans and those with low meat intake. The reported prevalence of B12 deficiency in early pregnancy is up to 70% in India. Women of South Asian ethnicity living in Vancouver demonstrated substantially lower vitamin B12 levels and higher rates of vitamin B12 deficiency and inadequacy in first trimester than women of European ancestry.66 Levels of B12 are lower in smokers, individuals with heavy alcohol intake, and in obese women.67 Low B12 is seen in more than 10% pregnant women with previous bypass bariatric surgery or sleeve gastrectomy.68 The long-term (>2 years) use of metformin may induce biochemical vitamin B12 deficiency, though whether this correlates with anemia or neurological complications is unclear. The prevalence of vitamin B12 deficiency in metformin-treated type 2 diabetic patients in a tertiary institution in Nigeria was 41%.69 Metformin used in the treatment of gestational diabetes mellitus did not result in lower holotranscobalamin levels than in insulin-treated women.70
B12 deficiency may manifest as maternal neurological or hematological complications, or as growth restriction, hypotonia, NTDs or loss of neuromotor skills in the neonate.71 Folate treatment may mask B12 deficiency by correcting megaloblastic anemia, while allowing neurological complications to progress. Thus, B12 levels should always be measured before commencing folate therapy.
Serum total vitamin B12 falls significantly in 20% of healthy women in pregnancy due to reduction in holohaptocorrin.72 Holotranscobalamin (active B12), the fraction of B12 that is available to cross the placenta, does not change and should be used as a guide to B12 deficiency in pregnancy.73 Methylmalonic acid (MMA) is a more specific functional indicator of B12 status than homocysteine, as the latter is also determined by folate and other B-vitamins.74 MMA and homocysteine assays are more expensive than B12 assays. Vitamin B12 levels vary significantly between different ethnicities, thus reference intervals during pregnancy need to be population and laboratory specific. A mobile POCD for measuring B12 was limited by a significant incubation time (3 h), multiple pipetting steps, and requirements for off-chip blood sample processing.75
The dietary requirement for vitamin B12 is 2.6 mg/day during pregnancy, and 2.8 mg/day during lactation.
B12 therapy may be administered orally, sublingually, intranasally, transdermally or parenterally. In women treated with oral cobalamin 1000 mg/day serum levels should be monitored to ensure adequate replacement. Sublingual therapy was more effective in increasing vitamin B12 levels than the standard intramuscular regimen.76 A daily low dose (50 mg) sublingual regimen was more effective than a 2000 mg once-weekly regimen in improving vitamin B12 levels in those with marginal deficiency.77
OTHER ELEMENTS
Other elements which may be a factor in nutritional anemia include zinc, copper, magnesium, B group vitamins, and vitamins A, C and E.78
Zinc requirements increase by 40% during pregnancy. The recommended daily allowance (RDA) is 11 mg per day, only 25% of which is absorbed. It is estimated that 25% of the world population is at risk of inadequate zinc intake, and deficiency is common in South Asia and Sub-Saharan Africa. Diets high in phytates and/or low in animal protein provide limited amounts of zinc. Zinc deficiency may also occur as a result of alcoholism, reduced absorption (celiac disease, bariatric surgery), gastrointestinal loss (Crohn’s disease), and diuretic therapy. Zinc is an important co-factor in iron metabolism, and zinc deficiency aggravates IDA.79 Maternal zinc deficiency may also be associated with miscarriage, premature birth, intrauterine growth restriction, birth defects and neurological disorders.80 Point-of-care testing for serum zinc has been developed for the management of pediatric septic shock, giving a result in 10–20 minutes.81
Copper is involved in Hb synthesis, neurotransmission, iron oxidation and the formation of connective tissue disease.82 Copper deficiency may result in anemia, neutropenia, optic neuropathy, myelopathy, neuropathy and other neurologic dysfunction. Copper deficiency has been reported in 29–34% of pregnant women in India.83 Foods rich in copper include organ meats such as liver and kidney, shellfish, grains, nuts and seeds. The RDA for pregnancy is 1 mg per day. Causes of acquired copper deficiency include intake of excessive amounts of zinc and iron (which reduce copper absorption), previous upper gastrointestinal surgery, malabsorption syndromes, alcohol excess and malnutrition. Serum copper and ceruloplasmin levels rise approximately two-fold in healthy pregnancy compared with non-pregnant women.84 Twenty-four hour urine copper levels may be useful in the diagnosis of copper deficiency in pregnancy.
Hypomagnesemia (hypoMg) is common in pregnancy in developing countries and low-income communities. Maternal hypoMg has been reported in 40–60% of pregnant women in South Asia, and 57% of pregnant women in the Sudan.85 It is unclear as to whether hypoMg may represent a direct cause, a consequence of other disease processes, or an epiphenomenon in adverse pregnancy outcomes.86 Mg absorption occurs primarily in the ileum and colon. Mg intake depends on the Mg concentration in drinking water and foods. Green leafy vegetables, grain, cereal, nuts and legumes are rich in Mg. Intermittent concentrations of Mg are found in fruit, meat and fish, and low Mg concentrations are present in dairy products. Refining and cooking of foods result in significant loss of Mg. There is a physiological decline in serum Mg in healthy pregnancy from a preconception mean of 0.93 mmol/L to a nadir mean of 0.63 mmol/L in third trimester. Several studies have shown a joint effect between magnesium and iron intake in relation to anemia.87 The China Health and Nutrition survey found serum magnesium was inversely associated with anemia in men and women.88 A high intake of magnesium was associated with a lower prevalence of anemia. In Sudanese women in early pregnancy low magnesium and low ferritin were associated with anemia. Gestational magnesium deficiency may be associated with hematological and teratogenic damage.89 Magnesium-deprived rats manifest a microcytic anemia with intense reticulocytosis suggestive of hemolysis.90 A high-magnesium diet improved anemia in a murine model of β-thalassemia.91
Riboflavin (vitamin B2) deficiency is endemic in populations where the staple diet consists of rice and wheat, with minimal or no consumption of dairy products or meat. Vegans and vegetarians are at highest risk of deficiency. Vitamin B2 degrades in light, and is also lost by the boiling or cooking of vegetables and grains. Deficiency of riboflavin tends to occur in combination with deficiency of other B group vitamins. Symptoms and signs include normocytic normochromic anemia with reticulocytopenia, atrophic glossitis, dermatitis and cheilosis.92 Bone marrow examination revealed selective hypoplasia of erythroid precursors, and ferrokinetics showed marked reduction of incorporation of iron into red blood cells.
Pyridoxine (vitamin B6) deficiency is an uncommon cause of anemia in pregnancy. Vitamin B6 deficiency is typically associated with a hypochromic microcytic anemia, and should be considered where anemia in pregnancy is unresponsive to iron therapy.93 Two cases of vitamin B6 responsive megaloblastic anemia in pregnancy have also been reported.94
Vitamin A deficiency has been reported in 15% of pregnant African women and 24% of pregnant Egyptian women.95 Eight per cent of pregnant women in Africa have night blindness.96 Daily requirements for vitamin A increase during pregnancy, the RDA being 800 mg/day. Vitamin A deficiency was associated with a 1.8 times greater risk of anemia than women without vitamin A deficiency in Bangladesh.97 The mechanism of anemia with vitamin A deficiency is unclear, postulated mechanisms including effects on erythropoietin gene transcription, modulation of iron metabolism and anti-infective properties. Vitamin A supplementation has been shown to improve Hb concentrations and reduce maternal anemia in communities where vitamin A deficiency is common.98,99
Vitamin C deficiency has been reported in up to 80% of pregnant women in Nigeria, 68% of pregnant women in Uganda, 31% of parturients in Brazil, and 75% of adults in rural India.100,101,102 Symptoms and signs of vitamin C deficiency include arthralgia, myalgia, follicular hyperkeratosis, spontaneous bleeding, gum hyperplasia, mood disturbance, jaundice, fever and anemia. Adult scurvy should be particularly considered in the setting of spontaneous petechiae, ecchymoses and mucosal bleeding. Scurvy-associated anemia may be microcytic, normocytic or macrocytic, and may be associated with hemolysis.103,104 The findings on blood film and bone marrow biopsy do not help differentiate scurvy-related anemia from the other megaloblastic anaemias, and measurement of vitamin C level is the gold standard for establishing diagnosis.105
Vitamin E deficiency is rare, occurring predominantly in the setting of fat malabsorption, rare disorders of fat metabolism and premature birth. Individuals in developing countries are at greater risk of vitamin E deficiency due to poor nutritional status and higher prevalence of oxidative stressors such as HIV infection and malaria which accelerate vitamin E depletion.106 Vitamin E deficiency is characterized by peripheral neuropathy, ataxia and anemia. Vitamin E may have a role in preventing stress-induced premature erythrocyte lysis by prevention of oxidation of polyunsaturated fatty acids and thus stabilizing the red blood cell membrane.107 Vitamin E deficiency is common in many hereditary hemolytic anemias. Supplementation with vitamin E reduces hemolysis and improves Hb in individuals with sickle cell disease, thalassemia and glucose-6-phosphate dehydrogenase deficiency.108 Vitamin E supplementation may also have a role in improving anemia in premature infants and patients with chronic kidney disease.109,110 A preliminary study of 30 mildly anemic adults found vitamin E supplementation for 3 months increased Hb.111 Major dietary sources of vitamin E include vegetable oils, nuts, whole grains and green leafy vegetables. The RDA for α-tocopherol in pregnancy is 15 mg/day.
CONCLUSION
Nutritional anemia is extremely common in women of worldwide, particularly in developing countries. Women of reproductive age frequently have micronutrient deficiencies at the time of conception, which may be further exacerbated by the demands of pregnancy and the fetus. Prevention of nutrient inadequacies may be best achieved by multiple nutrient fortification of water supplies and dietary substances which are cheap and are consumed universally such as salt. Point-of-care diagnostic testing may have a significant role in the diagnosis of nutritional anemias in remote areas. Treatment of nutritional anemia must consider the optimal mode of delivery taking medication side-effects, adherence, cost, the frequency of delivery, need for patient travel and administration supervision into account.
PRACTICE RECOMMENDATIONS
- Nutritional anemia in women of reproductive age is highly prevalent, particularly in Africa and South-East Asia.
- Iron deficiency is the most common cause of anemia in pregnancy.
- Diagnostic thresholds for iron deficiency vary significantly.
- There is a high prevalence of non-adherence with oral iron therapy because of gastrointestinal side-effects.
- Significant reactions to intravenous iron in second and third trimester are extremely rare.
- More research is needed regarding the safety of intravenous iron infusions in first trimester.
- Microcytosis is not a good screening test for iron deficiency.
- Most individuals with anemia due to folate deficiency do not manifest a macrocytosis.
- Point-of-care devices may enable testing for micronutrient deficiencies in remote areas.
CONFLICTS OF INTEREST
Author(s) statement awaited.
Feedback
Publishers’ note: We are constantly trying to update and enhance chapters in this Series. So if you have any constructive comments about this chapter please provide them to us by selecting the "Your Feedback" link in the left-hand column.
REFERENCES
Organisation WH. World Health Organization, Worldwide prevalence of anaemia 1993–2005: WHO global database on anaemia, 2008. (2008, accessed 30.6.19). | |
Harika R, Faber M, Samuel F, et al. Micronutrient Status and Dietary Intake of Iron, Vitamin A, Iodine, Folate and Zinc in Women of Reproductive Age and Pregnant Women in Ethiopia, Kenya, Nigeria and South Africa: A Systematic Review of Data from 2005 to 2015. Nutrients 2017;9. DOI: 10.3390/nu9101096. | |
Bothwell TH. Iron requirements in pregnancy and strategies to meet them. Am J Clin Nutr 2000;72:257S-64S. DOI: 10.1093/ajcn/72.1.257S. | |
Cromwell C PM. Hematologic changes in pregnancy. Elsevier, 2018:2203–14.e2205. | |
Auerbach M, Abernathy J, Juul S, et al. Prevalence of iron deficiency in first trimester, nonanemic pregnant women. J Matern Fetal Neonatal Med 2019:1–4. DOI: 10.1080/14767058.2019.1619690. | |
Joshi K, Nair S, Khade C, et al. Early gestation screening of pregnant women for iodine deficiency disorders and iron deficiency in urban centre in Vadodara, Gujarat, India. J Dev Orig Health Dis 2014;5:63–8. DOI: 10.1017/S2040174413000470. | |
Demmers MW, Niens M, van der Haar G, et al. Functional iron deficiency markers are absent during pregnancy despite evidence of low iron stores. Ann Clin Biochem 2019:4563219837290. DOI: 10.1177/0004563219837290. | |
Auerbach M. Commentary: Iron deficiency of pregnancy – a new approach involving intravenous iron. Reprod Health 2018;15:96. DOI: 10.1186/s12978–018–0536–1. | |
Koenig MD, Tussing-Humphreys L, Day J, et al. Hepcidin and iron homeostasis during pregnancy. Nutrients 2014;6:3062–83. DOI: 10.3390/nu6083062. | |
Jolobe OMP. Caveats in the work-up of iron deficiency anaemia. Eur J Intern Med 2018;48:e38. DOI: 10.1016/j.ejim.2017.07.032. | |
Petrosyan I, Blaison G, Andres E, et al. Anaemia in the elderly: an aetiologic profile of a prospective cohort of 95 hospitalised patients. Eur J Intern Med 2012;23:524–8. DOI: 10.1016/j.ejim.2012.03.013. | |
Francis J, Sheridan D, Samanta A, et al. Iron deficiency anaemia in chronic inflammatory rheumatic diseases: low mean cell haemoglobin is a better marker than low mean cell volume. Ann Rheum Dis 2005;64:787–8. DOI: 10.1136/ard.2004.025890. | |
Infusino I, Braga F, Dolci A, et al. Soluble transferrin receptor (sTfR) and sTfR/log ferritin index for the diagnosis of iron-deficiency anemia. A meta-analysis. Am J Clin Pathol 2012;138:642–9. DOI: 10.1309/AJCP16NTXZLZFAIB. | |
Zaman B, Rasool S, Jasim S, et al. Hepcidin as a diagnostic biomarker of iron deficiency anemia during pregnancy. J Matern Fetal Neonatal Med 2019:1–241. DOI: 10.1080/14767058.2019.1635112. | |
Jaggernath M, Naicker R, Madurai S, et al. Diagnostic Accuracy of the HemoCue Hb 301, STAT-Site MHgb and URIT-12 Point-of-Care Hemoglobin Meters in a Central Laboratory and a Community Based Clinic in Durban, South Africa. PLoS One 2016;11:e0152184. DOI: 10.1371/journal.pone.0152184. | |
Olatunya O, Ogundare O, Olaleye A, et al. Point-of-Care Testing for Anaemia in Children Using Portable Haematocrit Meter: A Pilot Study from Southwest Nigeria and Implications for Developing Countries. Ethiop J Health Sci 2016;26:251–8. DOI: 10.4314/ejhs.v26i3.8. | |
Srinivasan B, O'Dell D, Finkelstein JL, et al. ironPhone: Mobile device-coupled point-of-care diagnostics for assessment of iron status by quantification of serum ferritin. Biosens Bioelectron 2018;99:115–21. DOI: 10.1016/j.bios.2017.07.038. | |
Srinivasan B, Finkelstein JL, O'Dell D, et al. Rapid diagnostics for point-of-care quantification of soluble transferrin receptor. EBioMedicine 2019;42:504–10. DOI: 10.1016/j.ebiom.2019.03.017. | |
Kanuri G, Chichula D, Sawhney R, et al. Optimizing diagnostic biomarkers of iron deficiency anemia in community-dwelling Indian women and preschool children. Haematologica 2018;103:1991–6. DOI: 10.3324/haematol.2018.193243. | |
Martins JM. Universal iron fortification of foods: the view of a hematologist. Rev Bras Hematol Hemoter 2012;34:459–63. DOI: 10.5581/1516–8484.20120113. | |
Friesen V, Mbuya M, Neufeld L, et al. Fortified Foods Provide Varying Contributions to Iron, Vitamin a and Iodine Requirements of Women of Reproductive Age: Results from Coverage Surveys in 4 African Countries (P24–044–19). Curr Dev Nutr 2019;3. DOI: 10.1093/cdn/nzz044.P24–044–19. | |
Pachon H, Imhoff-Kunsch B, Shakya I, et al. Potential Dietary Contributions from Rice and Wheat Flour Fortification in the Solomon Islands: Results from the 2012–2013 Household Income and Expenditure Survey (P10–048–19). Curr Dev Nutr 2019;3. DOI: 10.1093/cdn/nzz034.P10–048–19. | |
Merryweather-Clarke AT, Pointon JJ, Shearman JD, et al. Global prevalence of putative haemochromatosis mutations. J Med Genet 1997;34:275–8. DOI: 10.1136/jmg.34.4.275. | |
Diosady LL, Mannar MGV, Krishnaswamy K. Improving the lives of millions through new double fortification of salt technology. Matern Child Nutr 2019;15(3):e12773. DOI: 10.1111/mcn.12773. | |
Mannar V, Diosady L. Quadruple Fortification of Salt with Iodine, Iron, Vitamins B9 and B12 to Reduce Maternal and Neonatal Mortality by Reducing Anemia and Nutritional Deficiency Prevalence (P24–041–19). Curr Dev Nutr 2019;3. DOI: 10.1093/cdn/nzz044.P24–041-19. | |
de Almeida CA, De Mello ED, Ramos AP, et al. Assessment of drinking water fortification with iron plus ascorbic Acid or ascorbic Acid alone in daycare centers as a strategy to control iron-deficiency anemia and iron deficiency: a randomized blind clinical study. J Trop Pediatr 2014;60:40–6. DOI: 10.1093/tropej/fmt071. | |
Rocha Dda S, Capanema FD, Netto MP, et al. Effectiveness of fortification of drinking water with iron and vitamin C in the reduction of anemia and improvement of nutritional status in children attending day-care centers in Belo Horizonte, Brazil. Food Nutr Bull 2011;32:340–6. DOI: 10.1177/156482651103200405. | |
de Oliveira JE, Scheid MM, Desai ID, et al. Iron fortification of domestic drinking water to prevent anemia among low socioeconomic families in Brazil. Int J Food Sci Nutr 1996;47:213–9. 1996/05/01. | |
Mehansho H. Iron fortification technology development: new approaches. J Nutr 2006;136:1059–63. DOI: 10.1093/jn/136.4.1059. | |
Casey GJ, Jolley D, Phuc TQ, et al. Long-term weekly iron-folic acid and de-worming is associated with stabilised haemoglobin and increasing iron stores in non-pregnant women in Vietnam. PLoS One 2010;5:e15691. DOI: 10.1371/journal.pone.0015691. | |
Organization WH. WHO e-library of evidence for nutrition actions 2019. Switzerland: WHO, 2019. | |
Casanueva E, Viteri FE, Mares-Galindo M, et al. Weekly iron as a safe alternative to daily supplementation for nonanemic pregnant women. Arch Med Res 2006;37:674–82. DOI: 10.1016/j.arcmed.2005.11.011. | |
Gluckman P HM, Seng CH, Bardsley A. Nutrition and Lifestyle for Pregnancy and Breastfeeding. Oxford University Press, 2015:1–430. | |
Jenkinson D. Single-dose intramuscular iron dextran in pregnancy for anaemia prevention in urban Zambia. J Trop Med Hyg 1984;87:71–4. | |
Auerbach M, James SE, Nicoletti M, et al. Results of the First American Prospective Study of Intravenous Iron in Oral Iron-Intolerant Iron-Deficient Gravidas. Am J Med 2017;130:1402–7. DOI: 10.1016/j.amjmed.2017.06.025. | |
Hallberg L, Ryttinger L and Solvell L. Side-effects of oral iron therapy. A double-blind study of different iron compounds in tablet form. Acta Med Scand Suppl 1966;459:3–10. | |
Rybo G, Solvell L. Side-effect studies on a new sustained release iron preparation. Scand J Haematol 1971;8:257–64. | |
Brock C, Curry H, Hanna C, et al. Adverse effects of iron supplementation: a comparative trial of a wax-matrix iron preparation and conventional ferrous sulfate tablets. Clin Ther 1985;7:568–73. | |
Tang M, Krebs NF. Update of pre- and postnatal iron supplementation in malaria endemic settings. Semin Perinatol 2019. DOI: 10.1053/j.semperi.2019.03.019. | |
Sangare L, van Eijk AM, Ter Kuile FO, et al. The association between malaria and iron status or supplementation in pregnancy: a systematic review and meta-analysis. PLoS One 2014;9:e87743. DOI: 10.1371/journal.pone.0087743. | |
Rogerson SJ, Meshnick S. Malaria in pregnancy: late consequences of early infections. J Infect Dis 2018. DOI: 10.1093/infdis/jiy738. | |
Komolafe JO, Kuti O, Ijadunola KT, et al. A comparative study between intramuscular iron dextran and oral ferrous sulfate in the treatment of iron deficiency anaemia in pregnancy. J Obstet Gynaecol 2003;23:628–31. DOI: 10.1080/01443610310001604394. | |
Govindappagari S, Burwick RM. Treatment of Iron Deficiency Anemia in Pregnancy with Intravenous versus Oral Iron: Systematic Review and Meta-Analysis. Am J Perinatol 2018. DOI: 10.1055/s-0038–1668555. | |
Qassim A, Gergis RG, Jeffries B, et al. Use of intravenous iron polymaltose in the management of iron deficiency in pregnancy: A retrospective cohort study. Aust N Z J Obstet Gynaecol 2018;58:163–9. DOI: 10.1111/ajo.12645. | |
Seeho SKM, Morris JM. Intravenous iron use in pregnancy: Ironing out the issues and evidence. Aust N Z J Obstet Gynaecol 2018;58:145–7. DOI: 10.1111/ajo.12794. | |
Froessler B, Gajic T, Dekker G, et al. Treatment of iron deficiency and iron deficiency anemia with intravenous ferric carboxymaltose in pregnancy. Arch Gynecol Obstet 2018. DOI: 10.1007/s00404–018–4782–9. | |
Qassim A, Mol BW, Grivell RM, et al. Safety and efficacy of intravenous iron polymaltose, iron sucrose and ferric carboxymaltose in pregnancy: A systematic review. Aust N Z J Obstet Gynaecol 2018;58:22–39. DOI: 10.1111/ajo.12695. | |
Weinberg ED. Can iron be teratogenic? Biometals 2010;23:181–4. DOI: 10.1007/s10534–009–9285–5. | |
Rodriguez RA MM, Alporta JPL, Luque CG, Mayor AC, Ruiz JA et al. Retrospective Case Reports of Anemic Pregnant Women Receiving Intravenous Ferric Carboxymaltose: Experience from a Tertiary Hospital in Spain. Obstetrics and Gynecology International 2016;2016:1–5. | |
Devasenapathy N, Singh R, Moodbidri P, et al. An Observational Study on the Use of IV Iron Sucrose Among Anaemic Pregnant Women in Government Healthcare Facilities from Two States of India. J Obstet Gynaecol India 2015;65:230–5. DOI: 10.1007/s13224–014–0588–2. | |
Avni T, Bieber A, Grossman A, et al. The safety of intravenous iron preparations: systematic review and meta-analysis. Mayo Clin Proc 2015;90:12–23. DOI: 10.1016/j.mayocp.2014.10.007. | |
Wolf M, Chertow GM, Macdougall IC, et al. Randomized trial of intravenous iron-induced hypophosphatemia. JCI Insight 2018;3. DOI: 10.1172/jci.insight.124486. | |
Abu Hashim H, Foda O, Ghayaty E. Lactoferrin or ferrous salts for iron deficiency anemia in pregnancy: A meta-analysis of randomized trials. Eur J Obstet Gynecol Reprod Biol 2017;219:45–52. DOI: 10.1016/j.ejogrb.2017.10.003. | |
Darwish AM, Fouly HA, Saied WH, et al. Lactoferrin plus health education versus total dose infusion (TDI) of low-molecular weight (LMW) iron dextran for treating iron deficiency anemia (IDA) in pregnancy: a randomized controlled trial. J Matern Fetal Neonatal Med 2018:1–7. DOI: 10.1080/14767058.2018.1429396. | |
Rateb A MA, Balsha K. The Effect of Orally Administered Iron-Saturated Lactoferrin on Systemic Iron Homeostasis in Pregnant Women Suffering from Iron Deficiency and Iron Deficiency Anaemia. The Egyptian Journal of Hospital Medicine 2018;71:2851–7. | |
Maurya A, Nanjappa SH, Honnavar S, et al. Rapidly Dissolving Microneedle Patches for Transdermal Iron Replenishment Therapy. J Pharm Sci 2018;107:1642–7. DOI: 10.1016/j.xphs.2018.02.011. | |
Murthy SN and Vaka SR. Irontophoresis: transdermal delivery of iron by iontophoresis. J Pharm Sci 2009;98:2670–6. DOI: 10.1002/jps.21641. | |
Modepalli N, Shivakumar HN, McCrudden MT, et al. Transdermal Delivery of Iron Using Soluble Microneedles: Dermal Kinetics and Safety. J Pharm Sci 2016;105:1196–200. DOI: 10.1016/j.xphs.2015.12.008. | |
Juluri A, Modepalli N, Jo S, et al. Minimally invasive transdermal delivery of iron-dextran. J Pharm Sci 2013;102:987–93. DOI: 10.1002/jps.23429. | |
Guo X, Zheng H, Guo Y, et al. Nasal delivery of nanoliposome-encapsulated ferric ammonium citrate can increase the iron content of rat brain. J Nanobiotechnology 2017;15:42. DOI: 10.1186/s12951–017–0277–2. | |
Rothman D. Folic acid in pregnancy. Am J Obstet Gynecol 1970;108:149–75. DOI: 10.1016/0002–9378(70)90221–8. | |
Bailey LB CM. Folate. In: Erdman JW MI, Zeisel SH (eds.) Present knowledge in nutrition. 10 edn. Washington: Wiley-Blackwell, 2012:321–42. | |
Frenkel EP, Yardley DA. Clinical and laboratory features and sequelae of deficiency of folic acid (folate) and vitamin B12 (cobalamin) in pregnancy and gynecology. Hematol Oncol Clin North Am 2000;14:1079–100, viii. 2000/09/27. | |
Rey EG, Finkelstein JL, Erickson D. Point-of-Care Assessment of Folate Status in Women of Reproductive Age Using a Fluorescence Lateral Flow Assay(). Conf Proc IEEE Eng Med Biol Soc 2018;2018:3906–9. DOI: 10.1109/EMBC.2018.8513377. | |
Moussa HN, Hosseini Nasab S, Haidar ZA, et al. Folic acid supplementation: what is new? Fetal, obstetric, long-term benefits and risks. Future Sci OA 2016;2:FSO116. DOI: 10.4155/fsoa-2015-0015. | |
Schroder TH, Sinclair G, Mattman A, et al. Pregnant women of South Asian ethnicity in Canada have substantially lower vitamin B12 status compared with pregnant women of European ethnicity. Br J Nutr 2017;118:454–62. DOI: 10.1017/S0007114517002331. | |
Sukumar N, Venkataraman H, Wilson S, et al. Vitamin B12 Status among Pregnant Women in the UK and Its Association with Obesity and Gestational Diabetes. Nutrients 2016;8. DOI: 10.3390/nu8120768. | |
Mead NC, Sakkatos P, Sakellaropoulos GC, et al. Pregnancy outcomes and nutritional indices after 3 types of bariatric surgery performed at a single institution. Surg Obes Relat Dis 2014;10:1166–73. DOI: 10.1016/j.soard.2014.02.011. | |
Owhin SO, Adaja TM, Fasipe OJ, et al. Prevalence of vitamin B12 deficiency among metformin-treated type 2 diabetic patients in a tertiary institution, South-South Nigeria. SAGE Open Med 2019;7:2050312119853433. DOI: 10.1177/2050312119853433. | |
Gatford KL, Houda CM, Lu ZX, et al. Vitamin B12 and homocysteine status during pregnancy in the metformin in gestational diabetes trial: responses to maternal metformin compared with insulin treatment. Diabetes Obes Metab 2013;15:660–7. DOI: 10.1111/dom.12080. | |
Bicakci Z. Growth retardation, general hypotonia, and loss of acquired neuromotor skills in the infants of mothers with cobalamin deficiency and the possible role of succinyl-CoA and glycine in the pathogenesis. Medicine (Baltimore) 2015;94:e584. DOI: 10.1097/MD.0000000000000584. | |
Milman N, Byg KE, Bergholt T, et al. Cobalamin status during normal pregnancy and postpartum: a longitudinal study comprising 406 Danish women. Eur J Haematol 2006;76:521–5. DOI: 10.1111/j.0902–4441.2006.t01–1-EJH2550.x. | |
Morkbak AL, Hvas AM, Milman N, et al. Holotranscobalamin remains unchanged during pregnancy. Longitudinal changes of cobalamins and their binding proteins during pregnancy and postpartum. Haematologica 2007;92:1711–2. DOI: 10.3324/haematol.11636. | |
Schroder TH, Tan A, Mattman A, et al. Reference intervals for serum total vitamin B12 and holotranscobalamin concentrations and their change points with methylmalonic acid concentration to assess vitamin B12 status during early and mid-pregnancy. Clin Chem Lab Med 2019. DOI: 10.1515/cclm-2018–1337. | |
Lee S, O'Dell D, Hohenstein J, et al. NutriPhone: a mobile platform for low-cost point-of-care quantification of vitamin B12 concentrations. Sci Rep 2016;6:28237. DOI: 10.1038/srep28237. | |
Bensky MJ, Ayalon-Dangur I, Ayalon-Dangur R, et al. Comparison of sublingual vs. intramuscular administration of vitamin B12 for the treatment of patients with vitamin B12 deficiency. Drug Deliv Transl Res 2019;9:625–30. DOI: 10.1007/s13346–018–00613-y. | |
Del Bo C, Riso P, Gardana C, et al. Effect of two different sublingual dosages of vitamin B12 on cobalamin nutritional status in vegans and vegetarians with a marginal deficiency: A randomized controlled trial. Clin Nutr 2019;38:575–83. DOI: 10.1016/j.clnu.2018.02.008. | |
Hercberg S, Rouaud C. Nutritional anaemia. Child Trop 1981:1–36. 1981/01/01. | |
Kelkitli E, Ozturk N, Aslan NA, et al. Serum zinc levels in patients with iron deficiency anemia and its association with symptoms of iron deficiency anemia. Ann Hematol 2016;95:751–6. DOI: 10.1007/s00277–016–2628–8. | |
Lewicka I, Kocylowski R, Grzesiak M, et al. Selected trace elements concentrations in pregnancy and their possible role – literature review. Ginekol Pol 2017;88:509–14. DOI: 10.5603/GP.a2017.0093. | |
Sukhavasi S, Jothimuthu P, Papautsky I, et al. Development of a Point-of-Care device to quantify serum zinc to aid the diagnosis and follow-up of pediatric septic shock. Conf Proc IEEE Eng Med Biol Soc 2011;2011:3676–9. DOI: 10.1109/IEMBS.2011.6090621. | |
Myint ZW, Oo TH, Thein KZ, et al. Copper deficiency anemia: review article. Ann Hematol 2018;97:1527–34. DOI: 10.1007/s00277–018–3407–5. | |
Gonmei Z, Toteja GS. Micronutrient status of Indian population. Indian J Med Res 2018;148:511–21. DOI: 10.4103/ijmr.IJMR_1768_18. | |
Vukelic J, Kapamadzija A, Petrovic D, et al. Variations of serum copper values in pregnancy. Srp Arh Celok Lek 2012;140:42–6. 2012/04/03. | |
Eltayeb R, Rayis DA, Sharif ME, et al. The prevalence of serum magnesium and iron deficiency anaemia among Sudanese women in early pregnancy: a cross-sectional study. Trans R Soc Trop Med Hyg 2019;113:31–5. DOI: 10.1093/trstmh/try109. | |
Morton A. Hypomagnesaemia and pregnancy. Obstet Med 2018;11:67–72. DOI: 10.1177/1753495X17744478. | |
Shi Z, Hu X, He K, et al. Joint association of magnesium and iron intake with anemia among Chinese adults. Nutrition 2008;24:977–84. DOI: 10.1016/j.nut.2008.05.002. | |
Zhan Y, Chen R, Zheng W, et al. Association between serum magnesium and anemia: china health and nutrition survey. Biol Trace Elem Res 2014;159:39–45. DOI: 10.1007/s12011–014–9967-x. | |
Hovdenak N, Haram K. Influence of mineral and vitamin supplements on pregnancy outcome. Eur J Obstet Gynecol Reprod Biol 2012;164:127–32. DOI: 10.1016/j.ejogrb.2012.06.020. | |
Piomelli S, Jansen V, Dancis J. The hemolytic anemia of magnesium deficiency in adult rats. Blood 1973;41:451–9. | |
De Franceschi L, Brugnara C, Beuzard Y. Dietary magnesium supplementation ameliorates anemia in a mouse model of beta-thalassemia. Blood 1997;90:1283–90. | |
Lane M, Alfrey CP, Jr. The Anemia of Human Riboflavin Deficiency. Blood 1965;25:432–42. | |
Hisano M, Suzuki R, Sago H, et al. Vitamin B6 deficiency and anemia in pregnancy. Eur J Clin Nutr 2010;64:221–3. DOI: 10.1038/ejcn.2009.125. | |
Aminu S BA, Suleiman A, Isah A, et al. Pyridoxine responsive megaloblastic anaemia in pregnancy: a case report of two patients. Highland Medical Research Journal 2008;6:1–2. | |
Hamdy AM, Abdel Aleem MM, El-Shazly AA. Maternal Vitamin A Deficiency during Pregnancy and Its Relation with Maternal and Neonatal Hemoglobin Concentrations among Poor Egyptian Families. ISRN Pediatr 2013;2013:652148. DOI: 10.1155/2013/652148. | |
Organization WH. Global Prevalence of Vitamin ADeficiency in Population at Risk: 1995–2005. 2009. | |
Ahmed F, Mahmuda I, Sattar A, et al. Anaemia and vitamin A deficiency in poor urban pregnant women of Bangladesh. Asia Pac J Clin Nutr 2003;12:460–6. | |
Muslimatun S, Schmidt MK, Schultink W, et al. Weekly supplementation with iron and vitamin A during pregnancy increases hemoglobin concentration but decreases serum ferritin concentration in Indonesian pregnant women. J Nutr 2001;131:85–90. DOI: 10.1093/jn/131.1.85. | |
van den Broek N, Dou L, Othman M, et al. Vitamin A supplementation during pregnancy for maternal and newborn outcomes. Cochrane Database Syst Rev 2010:CD008666. DOI: 10.1002/14651858.CD008666.pub2. | |
Ugwa EA, Iwasam EA, Nwali MI. Low Serum Vitamin C Status Among Pregnant Women Attending Antenatal Care at General Hospital Dawakin Kudu, Northwest Nigeria. Int J Prev Med 2016;7:40. DOI: 10.4103/2008–7802.176166. | |
Chiplonkar SA, Agte VV, Mengale SS, et al. Are lifestyle factors good predictors of retinol and vitamin C deficiency in apparently healthy adults? Eur J Clin Nutr 2002;56:96–104. DOI: 10.1038/sj.ejcn.1601291. | |
Madruga de Oliveira A, Rondo PH, Mastroeni SS, et al. Plasma concentrations of ascorbic acid in parturients from a hospital in Southeast Brazil. Clin Nutr 2008;27:228–32. DOI: 10.1016/j.clnu.2007.11.006. | |
Brown A. The anaemia of scurvy. Glasgow Med J 1951;32:95–109. | |
Shaikh H, Faisal MS and Mewawalla P. Vitamin C deficiency: rare cause of severe anemia with hemolysis. Int J Hematol 2019;109:618–21. DOI: 10.1007/s12185–018–02575-w. | |
Alhatem A, Cai D. Behind the Skin: A Rare Case of Scurvy-Associated Megaloblastic Anemia. Clin Med Insights Case Rep 2019;12:1179547619849036. DOI: 10.1177/1179547619849036. | |
Dror DK, Allen LH. Vitamin E deficiency in developing countries. Food Nutr Bull 2011;32:124–43. DOI: 10.1177/156482651103200206. | |
Elaroussi MA, Fattah MA, Meky NH, et al. Effects of vitamin E, age and sex on performance of Japanese quail. 1. Haematological indices and liver function. Br Poult Sci 2007;48:669–77. DOI: 10.1080/00071660701687688. | |
Jilani T, Iqbal MP. Does vitamin E have a role in treatment and prevention of anemia? Pak J Pharm Sci 2011;24:237–42. | |
Mydlik M, Derzsiova K, Racz O, et al. Antioxidant therapy by oral vitamin E and vitamin E-coated dialyzer in CAPD and haemodialysis patients. Prague Med Rep 2006;107:354–64. | |
Arnon S, Regev RH, Bauer S, et al. Vitamin E levels during early iron supplementation in preterm infants. Am J Perinatol 2009;26:387–92. DOI: 10.1055/s-0029–1214233. | |
Jilani T, Moiz B, Iqbal MP. Vitamin E supplementation enhances hemoglobin and erythropoietin levels in mildly anemic adults. Acta Haematol 2008;119:45–7. DOI: 10.1159/000115784. |
Online Study Assessment Option
All readers who are qualified doctors or allied medical professionals can now automatically receive 2 Continuing Professional Development credits from FIGO plus a Study Completion Certificate from GLOWM for successfully answering 4 multiple choice questions (randomly selected) based on the study of this chapter.
Medical students can receive the Study Completion Certificate only.
(To find out more about FIGO’s Continuing Professional Development awards programme CLICK HERE)
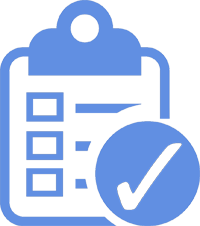